Two types of autologous cells in stricture development prevention after complete circular endoscopic dissection in minipig
Dva typy autologních buněk v prevenci vytvoření striktury po kompletní cirkulární endoskopické disekci u miniprasete
Úvod: Kompletní cirkulární endoskopická disekce (CED) je často doprovázena vytvořením pooperačních jícnových striktur. Pro prevenci těchto striktur byly nedávno testované rozličné typy terapeutických přístupů, např. buněčná terapie nebo jícnové stenty.
Metody: Pro studii byla použita miniaturní prasata Gottingen/Minnesota původu (n=10). Nejprve jsme ve středním jícnu provedli kompletní CED a poté byl defekt ponechán bez ošetření nebo pokryt suspenzí mezenchymálních kmenových buněk (MSCs) nebo kombinací MSCs a primárních orálních keratinocytů (pOKs) bez/s plně pokrývajícím samo-roztažným metalickým stentem (SEMS). Následně jsme provedli kontrolní endoskopii s extrakcí stentu a nekropsie byla provedena 17 až 36 dní po aplikaci buněk.
Výsledky: Všechny CED výkony byly dokončeny úspěšně bez závažných komplikací. Přestože jsme byli schopni detekovat MSCs nebo pOKs v post-CED defektech až do 36. dne po transplantaci, kombinace MSCs nebo MSCs/pOKs s nebo bez aplikace SEMS nezabránila vzniku jícnových striktur způsobených kompletní CED. Smíchání MSCs a pOKs mělo za následek vznik buněčných agregátů, které byly pozorovány převážně v submukóze a post-CED defekt byl pokryt tenkým jizvovitým epitelem obsahujícím kolagenová vlákna doprovázen rozličným stupněm rekonstrukce a integrity.
Závěr: Aplikace suspenze autologních MSCs samotných nebo v kombinaci s pOKs s nebo bez SEMS byla neefektivní v prevenci vzniku struktur po kompletní CED. Nicméně přítomnost MSCs nebo pOKs v defektu po CED byla potvrzena nejméně 5 týdnů po transplantaci.
Klíčová slova:
benigní striktura jícnu – cirkulární endoskopická disekce – endoskopická submukózní disekce – mezenchymální kmenové buňky – primární orální keratinocyty
Authors:
J. Juhásová 1; J. Klíma 1; J. Martínek 1,2; B. Walterová 1,3; R. Dolezel 1,4; Z. Vacková 1,2; M. Kollár 1,5; S. Juhas 1
Authors place of work:
Institute of Animal Physiology and Genetics, Czech Academy of Science, PIGMOD, Liběchov
1; Department of Hepatogastroenterology, Institute for Clinical and Experimental Medicine, Prague
2; Department of Surgery, 2nd Faculty of Medicine, Charles University and Central Military Hospital, Prague
4; Department of Clinical and Transplant Pathology, Institute for Clinical and Experimental Medicine, Prague
5; th Faculty of Medicine, Charles University, Prague
33
Published in the journal:
Rozhl. Chir., 2019, roč. 98, č. 12, s. 497-508.
Category:
Original article
doi:
https://doi.org/10.33699/PIS.2019.98.12.497–508
Summary
Introduction: Complete circular endoscopic dissection (CED) is frequently accompanied with post-operative strictures formation in the esophagus. Various types of therapeutic approaches have recently been tested to prevent these strictures, e.g. cell therapy or stenting.
Methods: Miniature pigs of Gottingen/Minnesota origin (n=10) were used in the study. First, we made the complete CED in the mid esophagus; next, the defect was left untreated or covered with mesenchymal stem cells (MSCs) or a mixture of MSCs and primary oral keratinocytes (pOKs) suspension without/with fully covered self-expandable metallic stent (SEMS). Consequently, we performed a control endoscopy with a stent removal, and necropsy was performed 17-36 days after cells application.
Results: All CED procedures were completed successfully without serious complications. Although we were able to detect MSCs or pOKs in the post-CED defects up to the 36th day after transplantation, the combination of MSCs or MSCs/pOKs with or without SEMS application did not prevent post-CED strictures development. The mixture of MSCs and pOKs resulted in the formation of cellular aggregates, which were mainly observed in submucosa, and the post-CED defect was covered with collagen fibers containing a thin scarred epithelium, accompanied by various degrees of reconstruction and integrity.
Conclusion: Suspension application of autologous MSCs alone or in combination with pOKs with or without SEMS was ineffective in the prevention of strictures formation after complete CED. Nevertheless, the presence of MSCs or pOKs in the post-CED defect was confirmed even 5 weeks after transplantation.
Keywords:
mesenchymal stem cells – benign esophageal stricture – circular endoscopic dissection – endoscopic submucosal dissection – primary oral keratinocytes
INTRODUCTION
Endoscopic submucosal dissection (ESD) or widespread endoscopic resection allows radical removal of circumferential or near-circumferential neoplastic esophageal lesions. The advantage of these endoscopic methods is their mini-invasivity and a low risk of major adverse events compared to traditional esophagectomy. The major drawback of these extensive resections is the development of strictures. The risk is 70–80% if more than 75% of the circumference is removed and almost 100% if the whole circumference is removed [1]. Some studies have shown that oral or local administration of steroids was a useful preventive option and significantly decreased stricture rates or the number of endoscopic balloon dilation (EBD) sessions [2–4]. On the other hand, high-dose pulse systemic methylprednisolone therapy for 3 days after ¾ or full circumferential ESD was ineffective in the prevention of esophageal stricture formation [5]. Recently new strategies have been tested to prevent post-ESD (non-/circumferential) esophageal strictures with different prevention effectivity, e.g. temporal application of esophageal stents alone or soaked with steroids, application of autologous mucosal patches [6–11]. Cell therapy alone or in combination with other treatments represents a promising approach for the prevention of stricture formation after circumferential ESD. The majority of esophageal stricture cell-based therapies used only one cell type (autologous oral epithelial cells, adipose derived stromal cells) applied as cell sheets in preclinical as well as clinical studies [12–20]. Recently, an orally administered conditioned medium from mesenchymal stem cells as gel has decreased the rate of strictures after semi-circumferential ESD in the pig model [21]. The goal of this preclinical experimental study was to estimate the fate of autologous pOKs and MSCs after their application into the post-ESD defect in the long-term period in a clinically acceptable and simplified manner as well as to detect their preventive effect (alone or in combination with a self-expandable metallic stent). However, an entirely effective method for the prevention of post-ESD stricture formation has not been found yet and no preventive method, despite partial effectivity, has gained a general acceptance to be used in clinical practice. Thus, a stricture prevention is still an unresolved issue, especially in patients undergoing a complete (full circumference) endoscopic submucosal dissection. The present study aimed to investigate whether the application of autologous mesenchymal stem cells (MSCs) or a mixture of MSCs and primary oral keratinocytes (pOKs) suspension can prevent stricture formation after circumferential esophageal ESD.
METHODS
Animals and anesthesia
All experiments were carried out according to the guidelines for the care and use of experimental animals and approved by the Resort Professional Commission of the CAS for Approval of Projects of Experiments on Animals (Approved Protocol No. 16/2017). Six miniature pigs (females, mean ± SD/52.33±7.39 kg/2.5±2.35 years old) from the Libechov breed (IAPG CAS, v.v.i.; PIGMOD; http://www.iapg.cas.cz/en/) were used for the experimental study. The minipigs were chosen as a suitable model given their considerable similarity to human anatomy, physiology and pathophysiology of the esophagus [22]. The animals were premedicated with intramuscular application of TKX mixture containing Tiletamine 4 mg/kg and Zolazepam 4 mg/kg (Zoletil 100, Virbac), Ketamine 10 mg/kg (Narketan 10, Chassot) and Xylazine 2 mg/kg (Rometar 2%, Spofa) as well as Atropine 0.05 mg/kg (BB Pharma a.s., Praha). After intravenous cannulation and intubation, Isoflurane (1.5%) combined with i.v. Fentanyl (3–5 ml/h) was used for inhalation anesthesia. The single-channel videogastroscope (GIF-Q165; Olympus, Tokyo, Japan) was used for all CED. After the procedure, the pigs received only liquid diet. Subsequently, control endoscopy followed by necropsy (depending on how the animals thrived) were done 17–36 days after the procedure.
Circular endoscopic dissection (CED)
First, coagulation marks were made to indicate the lower and upper margins of the incision (5 cm apart) in the middle esophagus. The lower circular incision was made, followed by the upper circular incision. Subsequently, both incisions were connected by endoscopic submucosal dissection and the en-bloc resection specimen was removed from the esophagus. Physiological solution with Patent blue V sodium salt (0.01%, 21605, Sigma-Aldrich) was used for submucosal injections to obtain adequate lifting, and atmospheric air was used for insufflation. Two types of electrocoagulation knives were used for the CED in the hybrid mode (30-W, monopolar): dual knife or IT knife (KD-650L, KD-611L; Olympus Medical Co, Tokyo, Japan) [23].
Experimental groups and stent application
Animals from group A received autologous mesenchymal stem cells (n=3; T79, T67, T83) and animals from group B obtained a mixture of mesenchymal stem cells and oral keratinocytes (n=3; L139, T61, L531). Fully covered, 103 mm long WallflexTM esophageal stents (O.D.=18 mm, REF: M00516700, Boston Scientific, USA) were used to cover the post-CED defects in one animal from both experimental groups (T79, L139). The prepared stents were applied under endoscopic control over the resection area, making sure that the stents exceed the lesion margins on both sides. The stents were fixed to the septum nasi (into the fibrotic part in front of the cartilage part) using a suspension technique with two polyamide threads (n=2) [23]. Animals (n=4; L586, N207, N145, P5) in control group were left untreated, with no stem cells and stent application.
Stem cells isolation, cultivation and tracking
Mesenchymal stem cells and oral keratinocytes were isolated 2–4 weeks before transplantation. Mesenchymal stem cells were isolated and cultivated as described in Juhasova et al. [24]. Buccal punches (Ø10mm) were stored in PBS at 4°C for maximum 5h until primary cell preparation. Autologous primary oral keratinocytes were isolated from buccal punches. Buccal tissue was sterilized by sequential washes in 10x Gentamycin solution in PBS for 10 minutes followed by 1x Antibiotic-Antimycotic solution (A-A, 15240-062, Thermo Fischer Scientific) in PBS for additional 10 min. Punches were cut into 1mm wide stripes and digested in 3ml Dispase solution (2.5 U/ml, 17105-041, Thermo Fisher Scientific) at 4°C, overnight. Next day epithelial sheets were mechanically detached under the binocular microscope. Pure sheets were trypsinized in 3ml trypsin/EDTA solution (R-001-100, Thermo Fisher Scientific) for 15 min at 37°C. Digestion was stopped with trypsin defined inhibitor (TDI, R007-100, Thermo Fisher Scientific) and the cell suspension was diluted by additional 5 ml of PBS. Epithelial cells were pelleted at 200 g for 10 min at room temperature and then re-suspended in complete EpiLife media with EDGS supplement (MEPI500CA and S0125, Thermo Fisher Scientific). The cells were seeded at the density of 2x104/cm2 on Coating matrix kit (R-001-K, Thermo Fisher Scientific) coated plastic flasks. The medium was changed every 2 days until 80% confluency. Apparent epithelial keratinocyte colonies were observed within one week. Two or three weeks later pOKs covered 80% surface of the flasks and were harvested and seeded at density of 20,000 cells/cm2. Generally, the cells were maintained for 4 weeks in culture. Primary oral keratinocytes were either cryopreserved in EpiLife medium with 10% DMSO or propagated until the 1st passage used for transplantation.
To track the transplanted cells, pOKs at the 1st passage and MSCs at the 2nd passage were labelled with yellow-green and red fluorescent LatexBeads beads (pOKs - L4655, MSCs - L3280; Sigma-Aldrich), respectively. Less than 80% confluent adherent cells were supplied with fresh culture media supplemented with 0.0005% solid content of latex beads and incubated at 37°C, 5% CO2 for 24 h prior to transplantation. Labelled cells in the cultivation plastic container were inspected and image recorded by the ChemiDoc™ XRS+ System (Bio-Rad Laboratories, USA) and Olympus IX73 Inverted fluorescence & phase microscope (Olympus, Japan).
Stem cells application
Before cell transfer, the cells were trypsinized, inhibited by TDI and washed in the media as above; cell count was determined via CountessTM II FL Automated Cell Counter (Thermo Fisher Scientific). 15–30 min before application, (mixed) cells were resuspended in 3 ml of transfer media consisting of 2% methylcellulose (medium viscosity, 21902, Sigma-Aldrich) in EpiLife basal media supplemented with 1x A-A and injected between the ESD lesion and the self-expandable metal stent (SEMS) or directly onto the ESD lesion (without SEMS application) using a standard ERCP endoscopic cannula, immediately after ESD creation (Tab. 1).
Endoscopy, necropsy, sample preparation, immunofluorescence and image acquisition
We performed control endoscopies on the 17th, 23rd and 36th day after CED. In two animals (L139 and T79), SEMS were extracted on the 23rd day after the procedure and the animals were euthanized 13 days later (36th day after CED). After post mortem measurement of esophageal strictures and adjacent tissue pathologies we photographed the samples macroscopically (Tab. 2) and immersed them into 4% paraformaldehyde in Phosphate Buffer Saline (PBS). After 24h immersion we transferred the tissue into 30% sucrose with sodium azide and left it there until perfect saturation. Then 10–20µm tissue sections were made using a cryostat (CM1950, Leica, Germany). For immunocytochemistry, tissue sections on glass were washed in PBS (3x5 minutes), successively treated with 10% goat serum (GibcoTM, 16210072) and 0.4% Triton® X-100 (#T8532, Sigma-Aldrich) in PBS for 1.5 hour at RT, primary antibodies (Anti-Cytokeratin 14 antibody [RCK107], #ab9220, Abcam, 1:250; MOUSE ANTI PIG MACROPHAGES [BA4D5], #MCA2317GA, Bio-Rad Antibodies, 1:500; Monoclonal Antibody to Cytokeratin (Pan-reactive) Phycoerythrin (PE) conjugated, #1P-108-C020, Exbio, 1:50) in 10% goat serum/0.4% Triton® X-100/PBS overnight (4°C), secondary antibody (Alexa Fluor® 647 goat anti-mouse IgG1 Cross-adsorbed, #A21240, Invitrogen, 1:500) in 10% goat serum/0.4% Triton® X-100/PBS for 1.5 hour at RT, and 4’,6-diamidino-2-phenylindole (#32670, Sigma-Aldrich) diluted 1:500 in PBS for 10 min at RT and mounted. Images of esophageal sections were obtained using a Leica SP5 confocal microscope equipped with 4 lasers (405, Argon, 561nm and 633nm lasers). Images were collected with a HCX PL APO lambda blue 40.0x1.25 OIL UV or HCX PL APO lambda blue 63.0x1.40 OIL UV objective. PMT setup, pinhole sizes (1 Airy) and contrast values were kept constant across different confocal sessions. Areas of analysis were z sectioned in 0.5- or 1-micron optical sections. Other serial tissue sections stained by Hematoxylin-Eosin (http://www.ihcworld.com/_protocols/special_stains/h&e_ellis.htm) were scanned by a virtual microscopy scanner (VS120-S5-fluorescence, Olympus). Images were collected with a UPLSAPO 40x/0.95 FN26.5 or UPLSAPO 20x/0.75 FN26.5 objective and the extent of the defect (µm) and re-epithelization (% of defect and height in µm) in ESD sites was measured using VS-ASW software (2.9 version, Olympus, Japan).
Tab. 2a
We detected the tracked cells using confocal microscopy (Fig. 3) and specific excitation and emission conditions (pOKs - λex ~470 nm and λem~505 nm; MSCs - λex~575nm and λem~610nm) as well as using brightfield microscopy by H&E staining (Fig. 4).
Phase contrast or fluorescence microscopy of primary oral keratinocytes from pig L139 (A or A´, 100x magnification), L531 (B or B´,
100x magnification) and T61 (C or C´, 200x magnification). Phase contrast or fluorescence microscopy of mesenchymal stem cells
from pig L139 (A´´ or A´´´, 100x magnification), L531 (B´´ or B´´´, 100x magnification), T61 (C´´ or C´´´, 200x magnification), T79 (D or
D´, 100x magnification), T67 (E or E´, 100x magnification) and T83 (F or F´, 100x magnification). Primary cell suspensions of animal
T61 prior to transplantation: bright field of MSCs and pOKs in both falcons (G), Cy3 fluorescence of mesenchymal stem cells in 50ml
falcon (G´), FITC fluorescence of labelled primary oral keratinocytes in 15ml falcon (G´´), images acquisition by the ChemiDoc™
XRS+ System.
Minipigs T61 (A), T67 (B), T83 (C), L531 (D), L139 (E) and T79 (F).
Fluorescent detection of primary oral keratinocytes (A2, 4, 5, green) and mesenchymal stem cells (A3, 4, 5, red) from pig L139 in
submucosa of the ESD site (A1 – DAPI, A4 merge of A1–3 channels, A5 merge of A2 and A3 channels, 630x magnification). Fluorescent
detection of primary oral keratinocytes (B2, 4, 5, green) and mesenchymal stem cells (B3, 4, 5, red) from pig L139 in cicatricial
mucosa of the ESD site (B1 – DAPI, B4 merge of B1–3 channels, B5 merge of B2 and B3 channels, 630x magnification). Fluorescent
detection of primary oral keratinocytes (C2, 4, 5, green) and mesenchymal stem cells (C3, 4, 5, red) from pig T61 in submucosa of
the ESD site (C1 – DAPI, C4 merge of C1–3 channels, C5 merge of C2 and C3 channels, 630x magnification). Fluorescent detection
of primary oral keratinocytes (D2, 4, 5, green) and mesenchymal stem cells (D3, 4, 5, red) from pig L531 in submucosa of the ESD
site (D1 – DAPI, D4 merge of D1–3 channels, D5 merge of D2 and D3 channels, 630x magnification). Fluorescent detection of
mesenchymal stem cells (E3, 4, red) from pig T79 in submucosa of the ESD site (E1 – DAPI, E4 merge of E1 and E3 channels, 400x
magnification). Fluorescent detection of mesenchymal stem cells (F3, 4, red) from pig T67 in submucosa of the ESD site (F1 – DAPI,
F4 merge of F1 and F3 channels, 400x magnification). Fluorescent detection of mesenchymal stem cells (G3, 4, red) from pig T83 in
submucosa of the ESD site (G1 – DAPI, G4 merge of G1 and G3 channels, 400x magnification).
CED defect of animal L139 with muscularis externa, a large portion of submucosa containing tracked pOKs and MSCs and thin cicatricial
mucosa two opposite arrowhead bounded (A1, scale=200μm; A1´detail from A1, scale=50μm; A1´´detail from A1´, scale=20μm;
A1´´´detail from A1´´, 400x magnification). Tracked pOKs and MSCs (black-brown particles, arrows) in submucosa and cicatricial mucosa
of animal L139 (scale=50μm). Tracked cells in submucosa of animal L531 (B), T61 (C), T67 (D), T79 (E) and T83 (F) (200x magnification).
Statistical analysis
The data are expressed as the means ± standard deviation (SD). The stenosis center diameter was compared using the Student’s t test. Probability values (P) of less than 0.05 were considered statistically significant. All statistical analyses were performed using Microsoft Excel (Microsoft Office 2013).
RESULTS
All CED procedures were performed successfully without any serious complications. Only in one animal (T61) slight perioperative bleeding was present that we stopped by endoscopic electrocoagulation. No visible perforations of the esophagus were observed during the procedures and no CED related pathologies were seen in the mediastinum at the time of necropsy. All CED procedures were made 40–55 cm from the snout end (approximately 5–20 cm orally from the cardia) to the extent of approximately 50 mm.
Proliferation and morphology of keratinocytes differed among the animals. While pOKs isolated from animals T61 (Fig. 1C) and L139 (Fig. 1A) formed tightly packed epithelial sheets on the plastic, animal L531 (Fig. 1B) formed sheets containing enlarged cells. Cell numbers corresponded to morphology; thus from animal L531 we harvested only 0.325x106 pOKs compared to animal L139 (5.32x106) and animal T61 (10.12x106) pOKs. Porcine MSCs showed spindle-shaped fibroblastic morphology as we previously reported [24] and the number of MSCs in all animals ranged from 36.7x106 (L531) to 57.35x106 (T61) (Fig. 1A´´, B´´, C´´). In animals T79, T67 and T83 (Fig. 1D, E, F) the MSCs morphology was similar to pOKs/MSCs animals.
Both pOKs and MSCs readily internalized fluorescent LatexBeads within only a 1-day incubation period as fluorescent beads were present in the cytoplasm of adherent cells under the microscope (Fig. 1A´, A´´´, B´, B´´´, C´, C´´´, D´, E´, F´). Free LatexBeads were washed during the cell harvesting and washing. Labelled cell suspensions were then checked for their fluorescence (Fig. 1G´, G´´) and mixed (pOKs + MSCs) before pelleting and re-suspending in the final 2% carboxymethylcellulose transfer medium (not shown).
Approximately 7–10 days after CED or SEMS removal (in two pigs) we observed clinical signs of esophageal stenosis in all animals (different intensity of emesis during food consumption or anorexia) which we confirmed during control endoscopies (Fig. 2). Animals were sacrificed under general anesthesia at control endoscopies on the 17th (T61, T67, T83), 23rd (L531) or 36th (L139, T79) day after CED or the 13th (L139, T79) day after SEMS removal.
When we calculated the stenosis center diameter (from circumference) mean in animals with transplantation of MSCs alone (including SEMS animal – T79), we detected a narrower stenosis (mean±SD=1.6±0.32 mm) compared to animals with transplantation of combined MSCs and pOKs (including one SEMS animal – L139; mean±SD=3.0±0.8 mm) but this difference was not significant (p=0.050248; t-test in Excel; Tab. 2). The stenosis center diameter mean in control animals (n=4) was 2.5±1.5 mm (mean±SD). There was no significant difference between the stenosis center diameters in control group animals and animals from group A (p=0.345084) and B (0.684951) (Tab. 2).
Marked autologous pig primary oral keratinocytes as well as mesenchymal stem cells were detected in all six animals using either fluorescence or H&E staining. Transplanted cells were mainly found in submucosa (100–800 µm from surface; Fig. 4A1, B, C, D, E, F) with occasional presence at the surface of the ESD defect site (Fig. 4A2, A3, A4). Only in animal L531, which received a minimal number of pOKs (325 000 cells) we detected occasional pOKs specific signals in tissue sections (Fig. 3D1–5, 4B). In all three animals with combined pOKs and MSCs application the cell suspensions resulted in the formation of aggregates that were detected histologically in submucosa and mucosa. Co-localization analysis revealed that some of the tracked pOKs were positive for cytokeratin 14 (Fig. 5D, D´) or pancytokeratin (Fig. 5C, C´). Interestingly, in animal T61 without SEMS application the mucosa-like layer of ESD site was strongly enriched by pancytokeratin positive cells (Fig. 5B1–5) and poor for macrophages (Fig. 5F) compared to animal L139 with SEMS application in which the analysis showed an opposite result (Fig. 5A1–5, E) whereas the time after CER or stent removal was similar (13th –17th days). In the ESD lesion site of all animals from group A and B we found a different portion of submucosa covered with thin cicatricial epithelium (26–230 µm) containing collagenous fibers showing various grades of reconstruction and integrity. Similar cicatricial epithelium was also found in ESD lesions of control animals with the range from 0 to 290 µm (mean±SD=105.0±115.0 µm; Tab. 3). In animal T79 with the metallic stent and MSCs application the individual parts of the ESD site showed the best re-structuralized epithelium with maximum high values (Tab. 3; Fig. 6A1–3).
Expression of pan-cytokeratin in post-CED defect submucosa close to cicatricial mucosa of animal L139 (A2, 5, purple) and T61
(B2, 5, purple) (A1, B1 – DAPI channel; A3, B3 – pOKs, green; A4, B4 – MSCs, red; A5, B5 – merge of A2, 3, 4 or B2, 3, 4 channels; 400x
magnification). Expression of pan-cytokeratin (purple) and its co-localization with pOKs/MSCs aggregates in submucosa of animal
L139 ESD site (C, C´, 400x magnification). Expression of cytokeratin 14 (purple) and its co-localization with pOKs/MSCs aggregates
in submucosa of animal L139 post-CED defect. (D, D´, 400x magnification). Expression of macrophage marker (purple) in post-CED
defect site submucosa close to cicatricial mucosa of animal L139 (E) and T61 (F, almost negative) (pOKs – green, MSCs – red, 400x
magnification).
The whole esophagus section in the post-CED defect site (A) which is delimited by the arrow (1.51x magnification). Details from
different parts of the CED defect with different grades of re-epithelization (A2>A1>A3>A4; A1, 3, 4=100x magnification; A2=50x
magnification).
DISCUSSION
Complete circular endoscopic dissection (CED) using the endoscopic submucosal dissection (ESD) technique represents a mini-invasive and effective method for the treatment of early neoplasia in the esophagus. However, stricture formation is the most frequent complication associated with healing after circumferential or near-circumferential esophageal ESD (= circular endoscopic dissection, CED). If more than 75% of esophageal circumference is removed, the risk of stricture development is about 80%; however, the risk of stricture is virtually 100% in patients with resection of the whole circumference (CED) [1]. Moreover, these strictures are often refractory requiring several sessions of endoscopic treatment. Thus, an effective prevention of post-CED strictures would be of great benefit for patients undergoing these extensive mucosal resections. Unfortunately, an effective preventive method has not been found yet. Our experimental study tested whether two types of autologous cells alone or combined with/without a clinically available self-expandable metallic stent would prevent esophageal strictures after CED. Unfortunately, mesenchymal stem cells application alone or in combination with primary oral keratinocytes (with or without stenting) failed to prevent strictures development. When we compared stricture severity between animals with transplantation of MSCs alone and animals with transplantation of combined MSCs and pOKs, we detected narrower strictures in the group with MSCs alone, but the difference was not statistically significant (compared to control group animals, as well) probably due to a low number of animals included. This fact might be caused by a partial beneficial action of pOKs to prevent esophageal strictures which was confirmed in several clinical and experimental studies [12,14–19]. On the other hand, in animal T79 with application of mesenchymal stem cells alone (with SEMS) we observed the best re-epithelization process among all experimental animals. This is in accordance with the results of other preclinical studies with application of adipose tissue-derived stromal cell (ADSC) as suspension [20] or cell sheets [13] in the canine or porcine model. Both those experimental studies showed a preventive effect of those stromal cells (mesenchymal stem cells) on esophageal strictures parameters after partial or fully circumferential ESD. Similarly, the preventive effect of MSC secretome on esophageal strictures formation was observed in the pig model [21].
Stricture severity was lowest in animal L139 (stenting and MSCs/pOKs) as the narrowest diameter was the widest (3.8 mm) among all experimental animals (with the exception of one control animal with 4.8 mm) – suggesting a positive stenting effect. Similarly, there were several studies declaring a preventive effect of different types of stents application in terms of esophageal stricture development after ESD in clinical studies [1,7,8,25,26].
Our study, in contrast to some other studies, did not find MSCs or MSCs/pOKs transplantation to prevent post-CED stricture development. However, in our study, all pigs underwent full circumferential endoscopic dissection while in most of the other studies, both clinical and experimental, the defect was not circumferential and cells transplantation alone is probably not sufficient to prevent stricture development after CED (in contrast to near-circumferential ESD). In two pigs, cells transplantation was accompanied by an insertion of SEMS but even this approach (transplantation + stent) did not prevent stricture formation. In pigs, SEMS need to be removed within three weeks because of severe tissue proliferation, while in humans, stents may be removed after 3 months. In pigs, hypergranulation and hypertrophic mucosa (Tab. 3) was created within few days after stent placement, maybe due to the more delicate and thinner structure of the esophagus in minipigs in comparison with the human esophagus.
Another problem occurring in our study was the retention of feed between the post-CED defect and SEMS, which probably affected the healing process. Uncovered muscle and submucosal tissue are unable to repair on their own and the post-CED defect is repaired per secundam intencionem. This fact corroborates our observation that most of the transplanted MSCs or pOKs sank into the submucosa, and we can only speculate how the rest of the tracked cells detected in cicatricial mucosa supported reparative processes after CED.
Our study has several limitation. The major limitation is the low number of experimental animals. However, as we could not reach our aim (stricture prevention), we decided not to include more animals into this study as it is unlikely that stricture prevention would have been achieved in subsequent animals. On the other hand, we were able to detect transplanted cells by two methodological methods (by fluorescence as well as by bright-field microscopy) and revealed their histological distribution in post-CED defects of all 6 animals after a long-term period (up to 36 days after implantation). In addition, our results suggest that combining MSCs or pOKs could be an interesting approach in future (maybe clinical) research. Finally, we could suggest performing cells transplantation in pigs with near-circumferential CED as this approach may actually be more effective in preventing strictures compared to CED.
CONCLUSION
Our experimental study supports the results of other studies, i.e. that the application of primary oral keratinocytes, mesenchymal stem cells or SEMS could have some beneficial effects in the prevention of esophageal stricture formation after CED, but these methods are unable to prevent stricture formation after CED. Our experiment revealed that transplanted cells applied in the form of simple cell suspensions were able to survive in both histological parts (submucosa and cicatricial mucosa) of the post-CED defect for a long period.
This work was supported by the grant NV16-27653A (Czech Health Research Council) – Prevention of esophageal strictures after endoscopic resection or dissection of early esophageal neoplasia-experimental study, the National Sustainability Program I, project number LO1609 (Czech Ministry of Education, Youth and Sports), and RVO: 67985904.
Conflict of interests
The authors declare that they have no conflict of interest in connection with this paper and that the article has not been published in any other journal.
MVDr. Štefan Juhás, PhD.
PIGMOD center
Laboratory of Cell Regeneration and Plasticity
Institute of Animal Physiology and Genetics
Czech Academy of Science v.v.i.
Rumburská 89
277 21 Liběchov
e-mail: juhas@iapg.cas.cz
Zdroje
- Martínek J, Juhas S, Dolezel R, et al. Prevention of esophageal strictures after circumferential endoscopic submucosal dissection. Minerva Chir. 2018;73:394–409. doi:10.23736/S0026-4733.18.07751-9.
- Yamaguchi N, Isomoto H, Shikuwa S, et al. Effect of oral prednisolone on esophageal stricture after complete circular endoscopic submucosal dissection for superficial esophageal squamous cell carcinoma: A case report. Digestion 2011;83:291–5. doi:10.1159/000321093.
- Isomoto H, Yamaguchi N, Minami H, et al. Management of complications associated with endoscopic submucosal dissection/endoscopic mucosal resection for esophageal cancer. Dig Endosc. 2013;25:29–38. doi:10.1111/j.1443-1661.2012.01388.x.
- Wang W, Ma Z. Steroid administration is effective to prevent strictures after endoscopic esophageal submucosal dissection: A network meta-analysis. Medicine (Baltimore). 2015;94(39):e1664–e1664. doi:10.1097/MD.0000000000001664.
- Nakamura J, Hikichi T, Watanabe K, et al. Feasibility of short-period, high-dose intravenous methylprednisolone for preventing stricture after endoscopic submucosal dissection for esophageal cancer: A preliminary study. Gastroenterol Res Pract. 2017. [On line]. doi: 10.1155/2017/9312517.
- Shi P, Ding X. Progress on the prevention of esophageal stricture after endoscopic submucosal dissection. Gastroenterol Res Pract. 2018. On line. doi: 10.1155/2018/1696849.
- Liao Z, Liao G, Yang X, et al. Transplantation of autologous esophageal mucosa to prevent stricture after circumferential endoscopic submucosal dissection of early esophageal cancer (with video). Gastrointest Endosc. Elsevier 2018;88:543–6. doi:10.1016/j.gie.2018.04.2349.
- Li L, Linghu E, Chai N, et al. Efficacy of triamcinolone-soaked polyglycolic acid sheet plus fully covered metal stent for preventing stricture formation after large esophageal endoscopic submucosal dissection. Dis Esophagus. 2018. [On line]. doi: 10.1093/dote/doy121.
- Chai N-L, Feng J, Li L-S, et al. Effect of polyglycolic acid sheet plus esophageal stent placement in preventing esophageal stricture after endoscopic submucosal dissection in patients with early-stage esophageal cancer: A randomized, controlled trial. World J Gastroenterol. 2018;24:1046–55. doi: 10.3748/wjg.v24.i9.1046.
- Shi K-D, Ji F. Prophylactic stenting for esophageal stricture prevention after endoscopic submucosal dissection. World J Gastroenterol. 2017;23:931–4. doi:10.3748/wjg.v23.i6.931.
- Yano T, Yoda Y, Nomura S, et al. Prospective trial of biodegradable stents for refractory benign esophageal strictures after curative treatment of esophageal cancer. Gastrointest Endosc. 2017;86:492–9. doi:10.1016/j.gie.2017.01.011.
- Yamaguchi N, Isomoto H, Kobayashi S, et al. Oral epithelial cell sheets engraftment for esophageal strictures after endoscopic submucosal dissection of squamous cell carcinoma and airplane transportation. Sci Rep. 2017;7:17460. doi: 10.1038/s41598-017-17663-w.
- Perrod G, Rahmi G, Pidial L, et al. Cell sheet transplantation for esophageal stricture prevention after endoscopic submucosal dissection in a porcine model.PLoS One. 2016;11:e0148249. doi: 10.1371/journal.pone.0148249.
- Ohki T, Yamato M, Ota M, et al. Prevention of esophageal stricture after endoscopic submucosal dissection using tissue-engineered cell sheets. Gastroenterology 2012;143:582–8.e2. doi:10.1053/j.gastro.2012.04.050.
- Ohki T, Yamato M, Murakami D, et al. Treatment of oesophageal ulcerations using endoscopic transplantation of tissue-engineered autologous oral mucosal epithelial cell sheets in a canine model. Gut 2006;55:1704–10. doi:10.1136/gut.2005.088518.
- Takagi R, Yamato M, Kanai N, et al. Cell sheet technology for regeneration of esophageal mucosa. World J Gastroenterol. 2012;18:5145–50. doi:10.3748/wjg.v18.i37.5145.
- Kanai N, Yamato M, Ohki T, et al. Fabricated autologous epidermal cell sheets for the prevention of esophageal stricture after circumferential ESD in a porcine model. Gastrointest Endosc. 2012;76:873–81. doi:10.1016/j.gie.2012.06.017.
- Kobayashi S, Kanai N, Tanaka N, et al. Transplantation of epidermal cell sheets by endoscopic balloon dilatation to avoid esophageal re-strictures: initial experience in a porcine model. Endosc Int Open. 2016;4:E1116–23. doi: 10.1055/s-0042-116145.
- Jonas E, Sjöqvist S, Elbe P, et al. Transplantation of tissue-engineered cell sheets for stricture prevention after endoscopic submucosal dissection of the oesophagus. United Eur Gastroenterol J. 2016;4:741–53. doi:10.1177/2050640616631205.
- Honda M, Hori Y, Nakada A, et al. Use of adipose tissue-derived stromal cells for prevention of esophageal stricture after circumferential EMR in a canine model. Gastrointest Endosc. 2011;73:777–84. doi:10.1016/j.gie.2010.11.008.
- Mizushima T, Ohnishi S, Hosono H, et al. Oral administration of conditioned medium obtained from mesenchymal stem cell culture prevents subsequent stricture formation after esophageal submucosal dissection in pigs. Gastrointest Endosc. 2017;86:542–52.e1. doi:10.1016/j.gie.2017.01.024.
- Vodička P, Smetana K, Dvořánková B, et al. The miniature pig as an animal model in biomedical research. Ann N Y Acad Sci. 2005;1049:161–71. doi:10.1196/annals.1334.015.
- Dolezel R, Walterova B, Juhas S, et al. Fixation of biomaterial to metallic stent and fixation of stents after circular endoscopic dissection in the esophagus on an animal model. Rozhl Chir. 2018;97:208–13.
- Juhásová J, Juhás Š, Klíma J, et al. Osteogenic differentiation of miniature pig mesenchymal stem cells in 2D and 3D environment. Physiol Res. 2011;60:559–71.
- Saito Y, Tanaka T, Andoh A, et al. Novel biodegradable stents for benign esophageal strictures following endoscopic submucosal dissection. Dig Dis Sci. 2008;53:330–3. doi:10.1007/s10620-007-9873-6.
- Wen J, Lu Z, Yang Y, et al. Preventing stricture formation by covered esophageal stent placement after endoscopic submucosal dissection for early esophageal cancer. Dig Dis Sci. 2014;59:658–63. doi:10.1007/s10620-013-2958-5.
Štítky
Chirurgie všeobecná Ortopedie Urgentní medicínaČlánek vyšel v časopise
Rozhledy v chirurgii
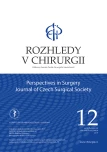
2019 Číslo 12
- Metamizol jako analgetikum první volby: kdy, pro koho, jak a proč?
- Neodolpasse je bezpečný přípravek v krátkodobé léčbě bolesti
Nejčtenější v tomto čísle
- Timing of cholecystectomy as the therapy for acute calculous cholecystitis
- Prehospital blood and blood products administration
- Treatment of liver injuries at the Trauma Centre of the University Hospital in Pilsen
- Cholangioscopy and intraductal ultrasonography in the diagnosis of cholangiocarcinoma