Prečo sú mitochondrie vhodné ciele pre liečbu rakoviny
Autoři:
Z. Tatarkova; S. Kuka; M. Petras; P. Racay; J. Lehotský; D. Dobrota; P. Kaplán
Působiště autorů:
Department of Medical Biochemistry, Jessenius Faculty of Medicine, Comenius University, Martin, Slovak Republic
Vyšlo v časopise:
Klin Onkol 2012; 25(6): 421-426
Kategorie:
Přehledy
Táto práca bola podporená projektom „Centrum excelentnosti pre výskum v personalizovanej terapii“ (CEVYPET), ITMS: 2622012053, spolufinancovaným zo zdrojov EÚ a Európskeho fondu regionálneho rozvoja, a grantom VEGA 1/0028/11 Ministerstva školstva a vedy Slovenskej republiky.
Obdrženo: 2. 12. 2011
Přijato: 31. 5. 2012
Autoři deklarují, že v souvislosti s předmětem studie nemají žádné komerční zájmy.
Redakční rada potvrzuje, že rukopis práce splnil ICMJE kritéria pro publikace zasílané do bi omedicínských časopisů.
Obdrženo: 2. 12. 2011
Přijato: 31. 5. 2012
Souhrn
Nové trendy v liečbe rakoviny sa spájajú s rozvojom presne cielených terapeutík, s účinkom na rakovinové bunky a zameraním na špecifické biologické dráhy. Úloha onkoproteínov a tumor-supresorových proteínov v proliferačnej signalizácii, regulácii bunkového cyklu a pozmenenej adhézii je už dobre preskúmaná. Chemické látky, vírusy a žiarenie sú tiež všeobecne prijímanými faktormi, ktoré vyvolávajú mutácie v génoch kódujúcich proteíny súvisiace s tvorbou rakoviny. Nedávne experimenty ukázali, že existujú dva nové kľúčové faktory pôsobiace na proliferujúce bunky – hypoxia a nedostatok glukózy. Tieto môžu iniciovať a podporovať proces malígnej transformácie v malom množstve buniek, ktorým sa podarilo uniknúť bunkovému starnutiu. Neregulovaná bunková proliferácia vedie k tvorbe bunkovej masy presahujúcej svoje rezervy, čo znižuje množstvo kyslíka a živín. Vzniknutý stav hypoxie iniciuje ďalšie kľúčové úpravy, ktoré umožňujú prežitie nádorových buniek. Proces apoptózy je potlačený a metabolizmus glukózy pozmenený. Nedávne experimenty naznačili, že vyčerpanie zásob kyslíka stimuluje mitochondrie, aby spracovávali väčšie množstvá reaktívnych foriem kyslíka (ROS). Aktivujú sa tak signálne dráhy, ako je hypoxiu-indukujúci faktor 1, ktoré podporujú prežívanie nádorových buniek a rast nádorov. Mitochondrie sú čoraz častejšie považované za kľúčové organely podieľajúce sa na chemoterapii, a preto je dôležité nájsť spôsob ako aktivovať apoptózu v mitochondriách za podmienok hypoxie, určiť vzťah medzi mitochondriami, ROS signalizáciou a procesmi aktivujúcimi prežívanie buniek. Každé nové zistenie môže otvoriť cestu pre pochopenie a odhalenie podstaty rakoviny a následné vytvorenie na mieru šitej terapie.
Kľúčové slová:
mitochondria – bunková smrť – energetický metabolizmus – bunková transformácia
Zdroje
1. Passos JF, Simillion C, Hallinan J et al. Cellular senescence: unravelling complexity. Age 2009; 31(4): 353–363.
2. Ralph SJ, Rodríguez-Enríquez S, Neuzil J et al. The causes of cancer revisited: mitochondrial malignancy and ROS-induced oncogenic transformation – why mitochondria are targets for cancer therapy. Mol Aspects Med 2010; 31(2): 145–170.
3. Muller FL, Lustgarten MS, Jang Y et al. Trends in oxidative aging theories. Free Radic Biol Med 2007; 43(4): 477–503.
4. Balaban RS, Nemoto S, Finkel T. Mitochondria, oxidants, and aging. Cell 2005; 120(4): 483–495.
5. Fleury C, Mignotte B, Vaysière JL. Mitochondrial reactive oxygen species in cell death signaling. Biochimie 2002; 84(2–3): 131–141.
6. Le Bras M, Clément MV, Pervaiz S et al. Reactive oxygen species and the mitochondrial signaling pathway of cell death. Histol Histopathol 2005; 20(1): 205–219.
7. Flores I, Blasco MA. The role of telomeres and telomerase in stem cell aging. FEBS Lett 2010; 584(17): 3826–3830.
8. Voghel G, Thorin-Trescases N, Mamarbachi AM et al. Endogenous oxidative stress prevents telomerase-dependent immortalization of human endothelial cells. Mech Ageing Dev 2010; 131(5): 354–363.
9. Houben JM, Moonen HJ, van Schooten FJ et al. Telomere length assessment: biomarker of chronic oxidative stress? Free Radic Biol Med 2008; 44(3): 235–246.
10. Klimova TA, Bell EL, Shroff EH et al. Hyperoxia-induced premature senescence requires p53 and pRb, but not mitochondrial matrix ROS. FASEB J 2009; 23(3): 783–794.
11. Aykin-Burns N, Ahmad IM, Zhu Y et al. Increased levels of superoxide and hydrogen peroxide mediate the differential susceptibility of cancer cells vs. normal cells to glucose deprivation. Biochem J 2009; 418(1): 29–37.
12. López-Lázaro M. A new view of carcinogenesis and an alternative approach to cancer therapy. Mol Med 2010; 16(3–4): 144–153.
13. Moreno-Sánchez R, Rodríguez-Enríguez S, Marín-Hernández A et al. Energy metabolism in tumor cells. FEBS J 2007; 274(6): 1393–1418.
14. Rodríguez-Enríguez S, Torres-Márquez ME, Moreno-Sánchez R. Substrate oxidation and ATP supply in AS-30D hepatoma cells. Arch Biochem Biophys 2000; 375(1): 21–30.
15. Gatenby RA, Gillies RJ. Why do cancers have high aerobic glycolysis? Nat Rev Cancer 2004; 4(11): 891–899.
16. Dang CV, Lewis BC, Dolde C et al. Oncogenes in tumor metabolism, tumorigenesis and apoptosis. J Bioenerg Biomembr 1997; 29(4): 345–354.
17. Marín-Hernández A, Rodríguez-Enríquez S, Vital-González PA et al. Determining and understanding the control of glycolysis in fast-growth tumor cells. Flux control by an over-expressed but strongly product-inhibited hexokinase. FEBS J 2006; 273(9): 1975–1988.
18. Semenza GL. Evaluation of HIF-1 inhibitors as anticancer agents. Drug Discov Today 2007; 12(19–20): 853–859.
19. Thomas DD, Espey MG, Ridnour LA et al. Hypoxic inducible factor 1, extracellular signal-regulated kinase, and p53 are regulated by distinct threshold concentrations of nitric oxide. Proc Natl Acad Sci USA 2004; 101(24): 8894–8899.
20. Guppy M. The hypoxic core: a possible answer to the cancer paradox. Biochem Biophys Res Commun 2002; 299(4): 676–680.
21. Robey IF, Lien AD, Welsh SJ et al. Hypoxia-inducible factor-1alpha and the glycolytic phenotype in tumors. Neoplasia 2005; 7(4): 324–330.
22. Simon MC. Coming up for air: HIF-1 and mitochondrial oxygen consumption. Cell Metab 2006; 3(3): 150–151.
23. Eskey CJ, Koretsky AP, Domach MM et al. Role of oxygen versus glucose in energy metabolism in a mammary carcinoma perfused ex vivo: direct measurement by 31P NMR. Proc Natl Acad Sci USA 1993; 90(7): 2646–2650.
24. Thews O, Kelleher DK, Lecher B et al. Blood flow, oxygenation, metabolic and energetic status in different clonal subpopulations of a rat rhabdomyosarcoma. Int J Oncol 1998; 13(2): 205–211.
25. Rofstad EK, Halsør EF. Vascular endothelial growth factor, interleukin 8, platelet-derived endothelial cell growth factor, and basic fibroblast growth factor promote angiogenesis and metastasis in human melanoma xenografts. Cancer Res 2000; 60(17): 4932–4938.
26. Schroeder T, Yuan H, Viglianti BL et al. Spatial heterogeneity and oxygen dependence of glucose consumption in R3230Ac and fibrosarcomas of the Fischer 344 rat. Cancer Res 2005; 65(12): 5163–5171.
27. Sutherland RM. Tumor hypoxia and gene expression – implications for malignant progression and therapy. Acta Oncol 1998; 37(6): 567–574.
28. Matoba S, Kang JG, Patino WD et al. p53 regulates mitochondrial respiration. Science 2006; 312(5780): 1650–1653.
29. Stubbs M, Bashford CL, Griffith JR. Understanding the tumor metabolic phenotype in the genomic era. Curr Mol Med 2003; 3(1): 49–59.
30. Pedersen PL. Tumor mitochondria and the bioenergetics of cancer cells. Prog Exp Tumor Res 1978; 22: 190–274.
31. LaNoue KF, Hemington JG, Ohnishi T et al. Defects in anion and electron transport in Morris hepatoma mitochondria. In: Hormone and Cancer. Mc Kerns KW (ed). New York: Academic Press 1974: 131–167.
32. Lichtor T, Dohrmann GJ. Oxidative metabolism and glycolysis in benign brain tumors. J Neurosurg 1987; 67(3): 336–340.
33. Zhu XL, Guppy M. Cancer metabolism: facts, fantasy and fiction. Biochem Biophys Res Commun 2004; 313(3): 459–465.
34. Griguer CE, Oliva CR, Gillespie GY. Glucose metabolism heterogeneity in human and mouse malignant glioma cell lines. J Neurooncol 2005; 74(2): 123–133.
35. Seyfried TN, Mukherjee P. Targeting energy metabolism in brain cancer: review and hypothesis. Nutr Metab 2005; 2: 30.
36. Hanahan D, Weinberg RA. The hallmarks of cancer. Cell 2000; 100(1): 57–70.
37. Zitvogel L, Apetoh L, Ghiringhelli F et al. Immunological aspects of cancer chemotherapy. Nat Rev Immunol 2008; 8(1): 59–73.
38. Morselli E, Galluzzi L, Kepp O et al. Nutlin kills cancer cells via mitochondrial p53. Cell Cycle 2009; 8(11): 1647–1648.
39. Gogvadze V, Orrenius S, Zhivotovsky B. Mitochondria in cancer cells: what is so special about them? Trends Cell Biol 2008; 18(4): 165–173.
40. Galluzi L, Morselli E, Kepp O et al. Mitochondrial gateways to cancer. Mol Aspects Med 2010; 31(1): 1–20.
41. Fantin VR, Berardi MJ, Scorrano L et al. A novel mitochondriotoxic small molecule that selectively inhibits tumor cell growth. Cancer Cell 2002; 2(1): 29–42.
42. Mason AR, Drummond MF. Public funding of new cancer drugs: Is NICE getting nastier? Eur J Cancer 2008; 45(7): 1188–1192.
43. Decaudin D, Castedo M, Nemati F et al. Peripheral benzodiazepine receptor ligands reverse apoptosis resistance of cancer cells in vitro and in vivo. Cancer Res 2002; 62(5): 1388–1393.
44. Toogood PL. Mitochondrial drugs. Curr Opin Chem Biol 2008; 12(4): 457–463.
45. Garattini R, Gianni M, Terao M. Retinoid related molecules an emerging class of apoptotic agents with promising therapeutic potential in oncology: pharmacological activity and mechanisms of action. Curr Pharm Des 2004; 10(4): 433–448.
46. Kang L, Wang ZY. Breast cancer cell growth inhibition by phenethyl isothiocyanate is associated with down-regulation of oestrogen receptor-α36. J Cell Mol Med 2010; 14(6B): 1485–1493.
47. Moon YJ, Brazeau DA, Morris ME. Dietary phenethyl isothiocyanate alters gene expression in human breast cancer cells. Evid Based Complement Alternat Med 2011; 2011: 1–8.
48. Wang XH, Cavell BE, Syed Alwi SS et al. Inhibition of hypoxia inducible factor by phenethyl isothiocyanate. Biochem Pharmacol 2009; 78(3): 261–272.
49. Yuan JM. Masonic Cancer Center at University of Minnesota. National Cancer Institute at the National Institutes of Health. Available from: http://cancer.gov/clinicaltrials/UMN-2007NT127.
50. Goldin J. Working the program. Moving through cancer: strength and fitness training improves outlook for cancer patients. Rehab Manag 2008; 134(2): 358–367.
51. Müller M, Siems W, Buttgereit F et al. Quantification of ATP-producing and consuming processes of Ehrlich ascites tumour cells. Eur J Biochem 1986; 161(3): 701–705.
52. Jaroszewski JW, Kaplan O, Cohen JS. Action of gossypol and rhodamine 123 on wild-type and multidrug-resistant MCF-7 human breast cancer cells: 31P nuclear magnetic resonance and toxicity studies. Cancer Res 1990; 50(21): 6936–6943.
53. Liu G, Kelly WK, Wilding G et al. An open-label, multicenter, phase I/II study of single-agent AT 101 in men with castrate-resistant prostate cancer. Clin Cancer Res 2009; 15(9): 3172–3176.
54. Liu H, Hu YP, Savaraj N et al. Hypersensitization of tumor cells to glycolytic inhibitors. Biochemistry 2001; 40(18): 5542–5547.
55. Simons AL, Ahmad IM, Mattson DM et al. 2-Deoxy-D-glucose combined with cisplatin enhances cytotoxicity via metabolic oxidative stress in human head and neck cancer cells. Cancer Res 2007; 67(7): 3364–3370.
56. Sweet S, Singh G. Accumulation of human promyelocytic leukemic (HL-60) cells at two energetic cell cycle checkpoints. Cancer Res 1995; 55(22): 5164–5167.
57. Armstrong JS, Hornung B, Lecane P et al. Rotenone-induced G2/M cell cycle arrest and apoptosis in a human B lymphoma cell line PW. Biochem Biophys Res Commun 2001; 289(5): 973–978.
58. Barrientos A, Moraes CT. Titrating the effects of complex I mitochondrial impairment in the cell physiology. J Biol Chem 1999; 274(23): 16188–16197.
59. Biasutto L, Dong LF, Zoratti M et al. Mitochondrially targeted anti-cancer agents. Mitochondrion 2010; 10(6): 670–681.
60. Rohlena J, Dong LF, Ralph SJ et al. Anticancer drugs targeting the mitochondrial electron transport chain. Antioxid Redox Signal 2011; 15(12): 2951–2974.
61. Dong LF, Jameson VJ, Tilly D et al. Mitochondrial targeting of vitamin E succinate enhances its pro-apoptotic and anti-cancer activity via mitochondrial complex II. J Biol Chem 2011; 286(5): 3717–3728.
62. Ralph SJ, Moreno-Sánchez R, Neuzil J et al. Inhibitors of succinate: quinone reductase/Complex II regulate production of mitochondrial reactive oxygen species and protect normal cells from ischemic damage but induce specific cancer cell death. Pharm Res 2011; 28(11): 2695–2730.
63. Parkin DM, Bray F, Ferlay J et al. Global cancer statistics, 2002. CA Cancer J Clin 2005; 55(2): 74–108.
64. Jemal A, Siegel R, Ward E et al. Cancer statistics, 2009. CA Cancer J Clin 2009; 59(4): 225–249.
Štítky
Dětská onkologie Chirurgie všeobecná OnkologieČlánek vyšel v časopise
Klinická onkologie
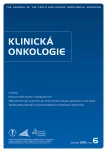
2012 Číslo 6
- Metamizol jako analgetikum první volby: kdy, pro koho, jak a proč?
- Cinitaprid – v Česku nová účinná látka nejen pro léčbu dysmotilitní dyspepsie
- Management pacientů s MPN a neobvyklou kombinací genových přestaveb – systematický přehled a kazuistiky
- Management péče o pacientku s karcinomem ovaria a neočekávanou mutací CDH1 – kazuistika
- Neodolpasse je bezpečný přípravek v krátkodobé léčbě bolesti
Nejčtenější v tomto čísle
- Hodnocení jaterní funkce v onkologické praxi
- Porovnání nákladů u režimů XELOX a FOLFOX-4 v léčbě kolorektálního karcinomu
- Bílé subkapsulární jaterní uzly objevené náhodně během laparoskopické operace: žlučové hamartomy a peribiliární žlázový hamartom
- Kazuistika: Podpůrná léčba u pacientky s rozsáhlým tumorem ovaria