APPLICATION OF TIME DOMAIN REFLECTOMETRY FOR CHARACTERIZATION OF HUMAN SKIN
In this paper, we report on time domain reflectometry (TDR) and transmission (TDT) measurement and characterization of skin using biosignal electrodes, normally used for monitoring of EMG biosignals. Basic principles of TDR and TDT methods are presented for various skin equivalent circuit configurations. Using the methods, the reflected and transmitted waves were measured at three different parts of the human body skin. The measured differences reveal differences in transient phenomena related to charging-discharging cells in a skin and underneath layers.
Keywords:
Time domain reflectometry, reflection, transmission, biosignal electrodes, transmission line
Authors:
Ľubomír Sládek 1; Alexander Šatka 1
Authors place of work:
Slovak University of Technology, Faculty of Electrical Engineering and Information, Institute of
Electronics and Photonics, Ilkovičova 3, 812 19, Bratislava, Slovak Republic
1
Published in the journal:
Lékař a technika - Clinician and Technology No. 2, 2012, 42, 23-26
Category:
Conference YBERC 2012
Summary
In this paper, we report on time domain reflectometry (TDR) and transmission (TDT) measurement and characterization of skin using biosignal electrodes, normally used for monitoring of EMG biosignals. Basic principles of TDR and TDT methods are presented for various skin equivalent circuit configurations. Using the methods, the reflected and transmitted waves were measured at three different parts of the human body skin. The measured differences reveal differences in transient phenomena related to charging-discharging cells in a skin and underneath layers.
Keywords:
Time domain reflectometry, reflection, transmission, biosignal electrodes, transmission line
Introduction
Many electric measurement methods can be used for the in vivo examination of the skin properties. These methods of human skin measurement allow the tracking of various skin affection or of the effect of therapy or cosmetic methods. Also tracking changes of these properties in time is very valuable.
Using electrical measurement methods, desired skin properties can be evaluated in frequency or time domain. Both are equally useful in electronic characterization, but the measurement in time domain gives direct information about signal propagation through its electrical path. In our work, transient analysis of biosignal electrode used for evaluation of skin properties and EMG monitoring was our main point of interest. This characterization is required due to optimal design of analog matching network of electronic EMG monitoring system and evaluation of skin properties using TDR.
Time Domain Reflectometry
TDR is measuring method which evaluates the step response of electronic devices or circuits to the voltage step in time domain. In comparison to other measurement techniques, time domain reflectometry provides direct view at the device under test (DUT) characteristics. Equipment (Fig. 1) used for this type of measurement consists of a step generator, an oscilloscope and transmission line ended by measured device. Using ultrafast step generator, fast leading or trailing edge is launched into the transmission line (TL) under investigation. As the incident wave (Ei) propagate trough the TL, reflected wave (Er) is generated by a load at the end of the line (Fig. 1a) [1].
Character of the reflected wave, sampled by the sampling circuit of a high speed oscilloscope shunt, resistive, capacitive, or inductive). Similarly, part of the incident wave transmits through the measured object. Evaluating transmitted wave (Et) gives additional information about transmission properties of the load (Fig. 1b).
Analyzing Reflections
Schematic of the TL with characteristic impedance Z0 ended by load impedance ZL and the basic relationship between Er and Ei is shown in Figure 2. In this Figure, there is an impedance discontinuity at the distance x at the TL. At this discontinuity, part of the incident wave is reflected, which can be quantitatively characterized by reflection coefficient ρ:
Shape of the reflections reveals nature and also magnitude of the mismatch. Figure 3 shows four typical reflected waves and the load impedance responsible for each. Assuming Z0 is real it is seen that resistive mismatches reflect a voltage of the same shape as the driving voltage, with the magnitude and polarity of Er determined by the relative values of Z0 and RL. These reflections are of interest for evaluation of load connection to the TL, e.g. electrical length of the connection to the tested device or impedance mismatch at the connectors etc.
In our case, main focus of our interests is the reflections produced by complex load impedances of biosignal electrodes. Examples of these reflections for four basic loads are depicted in Figure 4. In case of inductive load, impedance of inductor is high at the beginning, so part of incident wave is reflected back to input of TL, but as the impedance decreases, the inductor acts like short circuit on TL. In case of capacitive load, capacitor represents a short circuit on TL in first moment and as the capacitor charges, its impedance is rising and the rest of energy of incident pulse is reflected back to input of TL. The most interesting case which corresponds to our skin measurements is depicted in Figure 4b and lately explained in part with results.
Experimental setup
The setup for TDR measurement features a digital serial analyzer DSA-8200 and TDR sampling modules 80E02 manufactured by Tektronics. In this configuration, TDR measurement system allows truedifferential TDR measurements up to 30 GHz bandwidth with 12 ps incident rise time and 15 ps sampling time of reflected waves.
Biosignal electrodes are fabricated using printed circuit board (PCB) technology [5]. Their structure consists of FR-4 core material, conductive copper layer coated with gold, preventing electrode surface degradation due to the aggressive sweat. Topology and geometry of the electrodes is depicted in Fig. 5 [6], [7].
Connection of the electrodes to the measurement system is realized by BNC connectors and coaxial cables with characteristic impedance of 50Ω.
Results
Example of measured reflected and transmitted waves at three different places of human body (see Fig.6) are shown in Figure 7 and Figure 8. In all three traces, the reflected wave comprised of fast negative transient (see Figure 7a) corresponding to a capacitive short and a negligible low serial resistance, followed by a nearly exponential increase of the signal above the incident value. This corresponds to the situation depicted in Fig. 4b, where resistive component represents conductivity of the skin and capacitance component represent charging of the electrolyte at the cells.
Similarly, transmitted wave (Fig. 8) comprised of a fast, very short peak, corresponding to a serial capacitive short and a negligible low serial resistance between electrodes, followed by charging of the electrolyte at the cells and a slow relaxation to a steady state, where skin serial resistance dominates.
Using this information, the equivalent electrical circuit of skin measurements using TDR and TDT methods can be created, as depicted in Figure 9. Parameters of R1, C1 and R3, C3 can be quantified separately from TDR measurements, whereas R2, C2 from TDT measurements.
Conclusion
In conclusion, we have found that TDR is a reliable technique for characterization of different skin properties at different places of a human body. In addition to other techniques it allows direct observation and quantification of transient phenomena related to charging-discharging cells in a skin and underneath layers. In conclusion, TDR offers new insight into the characterization of skin properties using biosignal electrodes.
Acknowledgement
This work was supported by the Slovak Research and Development Agency under the contract No. VMSP-II- 0018-09 and under the contract No. VMSP-II-0016-09.
Zdroje
[1] Strickland, J., A., Zimmerman, H., A., Long, G., Frye, G., Time-domain reflectometry measurements, Tektronix, Inc., 1970
[2] “Time Domain Reflectometry Theory”, Application Note 1304-2, Agilent Technologies, Inc., May 10, 2006, 5966-4855E
[3] “Signal Integrity Analysis Series”, Application Note, Agilent Technologies, Inc., February 21, 2007, 5989-5763EN
[4] Andrews, J., R., Step Response and “S” Parameter Measurements in the Time Domain, Picosecond Pulse Labs, Application Note AN-3044, Revision 1, 5/89, May, 1989 [5] Vavrinský, E., Stopjaková, V., Brezina, I., Majer, L., Soláriková, P., Tvarožek, V., Electro-Optical Monitoring and Analysis of Human Cognitive Processes. In: Semiconductor Technologies. - Vukovar : InTech, 2010. ISBN 978-953-307- 080-3
[6] Vavrinský, E., Daříček, M., Donoval, M., Rendek, K., Horínek, F., Horniak, M., Donoval, D., Design of EMG Wireless Sensor System. In: Applied Electronics 2011: International Conference on Applied Electronics. Pilsen, 7-8 September 2011. Pilsen, University of West Bohemia, 2011. ISBN 978-80-7043-987-6 [7] Horínek, F. - Daříček, M. - Horniak, M. - Donoval, M. - Vavrinský, E. - Rendek, K. - Donoval, D.: Modulárny systém pre rýchlu diagnostiku ochorení a zvyšovanie kvality zdravotnej starostlivosti. In: EE Journal: Journal of Electrical Engineering and Energy. Vol. 16, p. 165-167, 2010. ISSN 1335-2547
Štítky
BiomedicínaČlánek vyšel v časopise
Lékař a technika
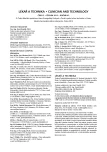
2012 Číslo 2
Nejčtenější v tomto čísle
- MECHANICAL MODEL OF THE CARDIOVASCULAR SYSTEM: DETERMINATION OF CARDIAC OUTPUT BY DYE DILUTION
- MATLAB AND ITS USE FOR PROCESSING OF THERMOGRAMS
- VENTILATOR CIRCUIT MODEL FOR OPTIMIZATION OF HIGH-FREQUENCY OSCILLATORY VENTILATION
- The Fifth Biomedical Engineering Conference of Young Biomedical Engineers and Researchers