IDENTIFICATION OF MAGNETIC NANOPARTICLES BY SQUID BIOSUSCEPTOMETRIC SYSTEM
A method of non-invasive localization of magnetic nanoparticles injected in living animal is presented. Measuring the magnetic response of magnetic nanoparticles to AC magnetic field by using SQUID biosusceptometric system is investigated. Experimental measurements in vivo and in vitro show possibility of using this method as a non-invasive method of identification and distribution of magnetic nanoparticles in biological tissues of small animals.
Keywords:
magnetic nanoparticles, SQUID, superparamagnetism, experimental animal
Authors:
M. Škrátek; I. Šimáček; A. Dvurečenskij; A. Cigáň
Authors place of work:
Institute of Measurement Science, Slovak Academy of Sciences, Bratislava, Slovakia
Published in the journal:
Lékař a technika - Clinician and Technology No. 2, 2012, 42, 35-37
Category:
Conference YBERC 2012
Summary
A method of non-invasive localization of magnetic nanoparticles injected in living animal is presented. Measuring the magnetic response of magnetic nanoparticles to AC magnetic field by using SQUID biosusceptometric system is investigated. Experimental measurements in vivo and in vitro show possibility of using this method as a non-invasive method of identification and distribution of magnetic nanoparticles in biological tissues of small animals.
Keywords:
magnetic nanoparticles, SQUID, superparamagnetism, experimental animal
Introduction
In recent years the use of magnetic nanoparticles in medicine has been risen. There are attempts to use them in cancer diagnostics, direct treatment like hyperthermia or the use of nanoparticles for targeted drug delivery. Magnetic nanoparticles (MNP) with appropriate properties, which are focused to the treated organ by external magnetic field, seem to be very useful carriers for various genes [1, 2, 3]. For biocompatibility these MNP should be covered by appropriate polymers, such as polyetylenglycol, polyetylenimin, heparin. The covering, shape and size of MNP is crucial from the view of transport properties in the cells and their toxicity. Unsuitable MNP may cause inflammatory reaction in various organs and tissues.
Determination of the distribution of MNP in living organism is the first important issue to confirm the effects of magnetic nanoparticles and also to evaluate the possible untoward effects. In this study, SQUID biosusceptometric system (SBS) was used for magnetic nanoparticle detection test, performed by in and ex vivo scanning of Wistar rats to clarify the distribution of MNP in living organism for the pharmacokinetics analysis, like drug delivers.
Methods
SBS consists of the RF SQUID, 2nd-order gradiometer, electronic modules, movable bed and the magnetization system with Helmholtz coils generating the magnetic field in the direction of gradiometer axis.
AC magnetic field has the intensity of 240 A/m and the frequency of 2.8 Hz.
This frequency enables to achieve a low level of the disturbing signal, as the most significant disturbing components are the fluctuations of the DC geomagnetic field component and the low-frequency band components below ~ 1 Hz, and also enables to minimize the effects of eddy currents (induced in the walls of the shielded chamber - Faraday cage) influence. The gradiometer sensor is placed close (~1 cm) to the surface of measured object. The spectral sensitivity of the measuring system (for the gradiometer pickup coil with the diameter of 2.8×10- 2 m) is ~ 2×10-14 T Hz-1/2.
Magnetometric measurement of MNP solution was performed with the same SQUID gradiometric system, by using different magnetization system and compensation coil [4].
Results
Studied Fe3O4 nanoparticles were prepared by microemulsion method at Polymer Institute of SAS by prof. Ignác Capek. These superparamagnetic Fe3O4 MNP were coated with citric acid and the mean hydrodynamic particle diameter was ~ 35 nm. From the magnetization measurement, fig. 1, the mass magnetic susceptibility was determined, which was later used to determine the concentration of this MNP in the organs of the tested animal.
Fig. 2 documents the measured values of the output signal Upp, depending on the distance d of the cylindrical surface of the model, where cF is the concentration of MNP in the water filled model.
i) From the characteristics is clear that the active area of the measured object is located near the surface.
The level of the magnetic signal decreases with a ratio of approximately 1/d3, so it can be assumed that the effective detection of MNP will be in ∼ 5 cm depth. Sensing characteristic of the 2nd-order gradiometer is narrow, so effective detection field has a shape of cone with a base diameter of 5 cm and a height of 5 cm. On the other side, this narrow sensing characteristics allows better determining of different cF in various locations of the measured object.
ii) Living biological tissue is diamagnetic and this is presented on figures with negative values of output signal Upp. This means that at low values of cF, the measured object still will appear as diamagnetic. Specifically, for our SBS and the object with used 35 nm MNP solution, the transition from paramagnetic to diamagnetic state is expected when the value of cF is in the range 13 - 20 μgFe3O4 cm-3. Relationship Upp and cF is influenced mainly by contribution of diamagnetic biological tissues, which in terms of susceptibility appears to be water, paramagnetic MNP and also paramagnetic air layer between the sensor and the surface of the measured object.
Results of measurements of Upp in experimental animal, which was injected to tail with 1 cm3 solution of MNP and after 5 minutes measured, could be seen in fig. 3.
Measured Upp values clearly show the difference between the magnetic response before and after injection of the MNP solution. Plotted values of Upp correspond to cF obtained during movement of the animal under the SQUID sensor along the torso (left part) and upper part of the tail (right side). From the measured values, the accumulation of MNP in the region of heart and liver could be assumed.
Subsequently, the animals were sacrificed and selected organs were removed to be measured with the SBS. Obtained data were used to determine the magnetic susceptibility of tissues, or cF of MNP in the tissue. From the results shown in fig. 5, MNP are mostly accumulated in the liver.
Conclusion
In the paper SBS and the method for measurement of magnetic response of Fe3O4 MNP to the AC magnetic field in the samples and also of injected MNP to tissues of the living animal are presented. Dependences between measured output signal, the concentration and distance of the sensor from magnetized MNP were obtained. Experimental measurements on living rat showed a possibility of using this method as an non-invasive method of identification and distribution determination of MNP in biological tissues of small animals. It can be assumed that by focusation of MNP with the external magnetic field, MNP could be accumulated in other areas of the animal body.
Acknowledgement
This work was supported by Agency of the Ministry of Education of the Slovak Republic for the Structural Funds of the EU, Operational Programme Research and Development (OPVaV-2009/4.1/02-SORO), Project Code 26240120019 (0.7) and by VEGA grant 2/0160/10.
Mgr. Martin Škrátek, PhD.
Institute of Measurement Science
Slovak Academy of Sciences
Dúbravská cesta 9, SK-841 04 Bratislava
E-mail: martin.skratek@savba.sk
Phone: +421 259 104 528
Fax: +421 254 775 943
Zdroje
[1] M. L. Tan, P. F. Choong, C. R. Dass: Cancer, chitosan nanoparticles an catalytic nucleis acids, J. Pharm. Pharmacol. , 2009, vol. 61, p. 3-12.
[2] M. Koneracká, P. Kopčanský, P. Sosa, J. Bageľová, M. Timko: Interliposomal transfer of crystal violet dye from DPPC liposomes to magnetoliposomes, Journal of Magnetism and Magnetic Materials, 2005, vol. 293, p. 271-276.
[3] M. Koneracká, P. Kopčanský, M. Timko, C. N. Ramchand: Direct binding procedure of proteins and enzymes to fine magnetic particles, Journal of Magnetism and Magnetic Materials, 2002, vol. 252, p. 409-411.
[4] V. Zrubec: Improved system for measurement of the magnetization characteristics of the high-temperature superconductors by the compensation method in weak magnetic fields, Review of scientific instruments, 2001, vol. 72, no. 6, p. 2653-2660
Štítky
BiomedicínaČlánek vyšel v časopise
Lékař a technika
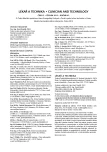
2012 Číslo 2
Nejčtenější v tomto čísle
- MECHANICAL MODEL OF THE CARDIOVASCULAR SYSTEM: DETERMINATION OF CARDIAC OUTPUT BY DYE DILUTION
- MATLAB AND ITS USE FOR PROCESSING OF THERMOGRAMS
- VENTILATOR CIRCUIT MODEL FOR OPTIMIZATION OF HIGH-FREQUENCY OSCILLATORY VENTILATION
- The Fifth Biomedical Engineering Conference of Young Biomedical Engineers and Researchers