Focus on perchlozone, an anti-tuberculosis drug from the Russian Federation
Authors:
Ivan Malík 1,2; Jozef Čižmárik 3; Mária Pecháčová 3
Authors‘ workplace:
Department of Pharmaceutical Chemistry, Faculty of Pharmacy, Comenius University in Bratislava Odbojárov 10, 832 32 Bratislava, Slovak Republic
1; Institute of Chemistry, Clinical Biochemistry and Laboratory Medicine, Faculty of Medicine, Slovak Medical University in Bratislava, Limbová 12, 833 03 Bratislava, Slovak Republic e-mail: malik@fpharm. uniba. sk
2; Department of Pharmaceutical Chemistry, Faculty of Pharmacy, Comenius University in Bratislava, Slovak Republic
3
Published in:
Čes. slov. Farm., 2020; 69, 203-210
Category:
Review Article
Overview
The prevalence of multidrug-resistant tuberculosis (MDR--TB) and extensively drug-resistant tuberculosis (XDR--TB) has been increasing at an alarming rate worldwide. Today’s “Fight against Tuberculosis“ programmes in the Russian Federation are subsidized by state and regional governments as well as health authorities. Each region has its own specific characteristics and needs specific interventions. Although some novel anti-tuberculosis (anti-TB) drugs (bedaquiline, delamanid) were approved by relevant authorities, and some promising compounds, especially those of oxazolidinones, are in various phases of clinical trials worldwide, the finding of effective, safe, pharmacokinetically favo-rable, economically and logistically accessible anti-TB agents still remains a serious challenge for medical and pharmaceutical sciences. Perchlozone, a compound containing a thiosemicarbazone scaffold, was approved in the Russian Federation in 2012 for the treatment (alone or as the active component of complex treatment regimens) of HIV-1 negative as well as HIV-1 positive patients suffering from MDR-TB or XDR-TB. Mechanism of anti-TB action of perchlozone might be similar to that of thiacetazone, which belongs into the same chemical class. Perchlozone has to be probably activated into reactive species by a mycobacterially encoded monoxygenase (EthA). The activated forms might act in multiple ways, including inhibition of mycobacterial cell wall synthesis due to interfence with a dehydration step of the type II fatty acid synthase pathway or sensitization of the Mycobacterium tuberculosis cell to oxidative stress. Favorable toxicological properties of perchlozone and its tolerability by the human organism were confirmed within revevant preclinical and clinical studies. However, recent preliminary investigations in vivo (animal models) could indicate genotoxicity after subacute inhalation of the drug. Regarding this issue, further development of more convenient nano- or microparticle-based formulations of perchlozone potentially improving targeted delivering and efficiency as well as decreasing (eliminating) its eventual toxicity might be taken into strong consideration.
Keywords:
perchlozone – Mycobacterium tuberculosis – resistance – MDR-TB – XDR-TB – HIV-1 co-infection
Introduction
Tuberculosis (TB) is a communicable airborne infectious disease. Studies of human skeletons showed that this potentially deadly disease plagued humankind for millennia but its cause remained unknown until 1882, when Dr. Robert Koch announced discovery of a bacillus subsequently termed Mycobacterium tuberculosis1, 2).
TB was regarded as one of relatively satisfactorily controlled infections for several decades, however, the emergence of drug-resistant tuberculosis (DR-TB) is becoming a major global threat3). Many forms of resistance of strains from Mycobacterium sp., including M. tuberculosis, to activity of drugs have been developed and can be found worldwide. The DR-TB form is caused by the Mycobacterium bacteria that are resistant to at least one first-line anti-TB drug, i.e., isoniazid (INH), rifampicin (RIF), pyrazinamide (PZA), or ethambutol (EMB). Polydrug-resistant TB (PDR-TB) indicates the resistance of the mycobacterial organisms to more than one anti-TB drug, but not including INH neither RIF. The MDR-TB disease is caused by the mycobacterial organisms resistant to at least INH and RIF. Original definition of extensively drug-resistant tuberculosis (XDR-TB) needs to be modified as all-oral regimens become the standard of care. Pre-extensively (pre-extremely) drug-resistant TB (pre-XDR-TB) is caused by the multidrug-resistant mycobacterial strains, which are, in addition, resistant to any fluoroquinolone (FQ) or second-line injectable agent, i.e., amikacin (AK), kanamycin (KAN), or capreomycin (CAP). The XDR-TB form is caused by the mycobacterial organisms, which show multidrug-resistance, and are resistant to any FQ and at least one of the second--line injectable anti-TB agents (AK, KAN, or CAP)4).
Increasing incidence of MDR-TB and XDR-TB worldwide is a major concern for TB control programs5–7). In 2018, there were about half a million new cases of RIF-resistant TB (RR-TB), of which 78% had MDR-TB. Countries with the largest share of global burden were India (27%), China (14%) and the Russian Federation (9%)3). Globally, 3.4% of new TB cases and 18% of previously treated cases had MDR-TB, or RR-TB, with the highest proportions (> 50% in previously treated cases) in the countries of the former Soviet Union3).
Regarding the fact that DR-TB notification rates relative to the population continue to increase in the Russian Federation despite the impressive decline in new TB notification rates8), next sections of the paper provide a very brief overview on global situation together with possible promising non-pharmacotherapetic and especially pharmacotherapeutic interventions, including characterization and practical experiences with one original anti-TB drug developed in this country.
Current prevalence of tuberculosis in the Russian Federation
The prevalence of TB in some regions of the Russian Federation was characterized by a “certain“ stabilization in the first decade of the 21st century. However, current overall situation remains very complicated and tense due to quite low level of prevention in adults together with worsening of clinical forms of both MDR-TB and XDR-TB9).
Today’s “Fight against Tuberculosis“ programmes are heavily subsidized by the state and regional governments as well as health authorities. The Russian Federation is one of the largest and most ethnically diverse multi-national federations in the world. The fact is that each region, i.e., particular federal subjects (republics, oblasts (provinces), krais (territories), autonomous okrugs (areas), autonomous oblasts, or federal cities) and Federal Okrugs (subdivided into city okrugs and municipal raions), of the Russian Federation has its own specific characteristics and needs specific interventions10–12).
Control of TB in some regions has been noticeably improved than in the others due to systematic and consistent implementation of fundamental principles of the TB control. The principles included rapid and reliable MDR-TB diagnostics, special chemotherapeutic strategy (including systematic registration of all TB cases, rehabilitation, multidrug chemotherapy schemes and provisions of support to overcome negative social stigma associated with the disease) as well as application of effective infection control practices9–12).
More favorable epidemiological profile of TB burden in Tomsk Oblast might be attributed to highly effective anti-DR-TB programme, which was implemented in this region only. The programme has been recognized as one of the most successful projects to manage MDR-TB in the world. In contast, overall burden of TB in the Asian part of the Russian Federation is still very serious. The regions, where interventions are needed most urgently, are Chukotka Autonomous Okrug, Jewish Autonomous Oblast and Tyva Republic as well9–12).
Continuous search for efficient and safe anti-tuberculosis drugs and vaccines
The aims of TB-treatment are to kill mycobacteria quickly, prevent the development and resistance to drugs, eliminate lasting mycobacteria and prevent relapse and transmission of the disease13), respectively. On the contrary, the tendency of mycobacteria to mutate and develop resistance to “simple“ treatment is very common and was the reason for combined treatment of MDR-TB and XDR-TB, respectively14). Classification of the compounds, which were recommended by the World Health Organization (WHO) for use in both MDR-TB and XDR--TB regimens, can be found in reviews6, 7).
In recent years, only a few effective drugs were approved and released for clinical practice in order to treat patients suffering from MDR-TB, XDR-TB, treatment-intolerant or non-responsive MDR-TB as a part of combination regimen14–17).
Bedaquiline (BDQ; 1), a molecule containing a diarylquinoline scaffold, delamanid (DLM; 2), a nitro-dihydro-imidazooxazole derivative, and pretomanid (PTM; 3), in whose structure a nitroimidazooxazine moiety is incorporated, represent new anti-TB agents. These compounds were approved by relevant authorities for using in clinical practice in different countries nearly 60 years after the last approval and release of a “classical“ RIF. Two modern drugs, BDQ (1) and DLM (2), have been also registered in the Russian Federation14–19).
The diarylquinoline derivative, BDQ (1) (Fig. 1), inhibits ATP generation in M. tuberculosis by interfering with its F-ATP synthase activity. Two mechanisms of action are broadly established for this molecule. Firstly, direct mechanism involves compound’s binding to enzyme’s c-ring to block its rotation, thus inhibiting ATP synthesis in enzyme’s catalytic α3β3-headpiece. The process leads to depletion of bacterial ATP20). Secondly, indirect mechanism involves BDQ’s (1) uncoupling electron transport in the electron transport chain from ATP synthesis at the F-ATP synthase21). However, given uncoupler mechanism was not confirmed by recent findings of Sarathy et al. (2019)22).
Another anti-TB agent approved recently, DLM (2) (Fig. 2), inhibits synthesis of mycobacterial cell wall components, methoxymycolic acid and ketomycolic acid. Mycolic acids are a complex mixture of branched, long-chain (C60–C90) fatty acids, representing key components of the highly hydrophobic cell wall23). The compound (2) is a prodrug, which gets activated by a specific enzyme, deazaflavin dependent nitroreductase (Rv3547). The reactive intermediate metabolite, formed between DLM (2) and the desnitro-imidazooxazole derivative, is considered to play a vital role in the inhibition of mycolic acid production24).
Stover et al. (2000) suggested25) that PTM (3) (Fig. 3) could act on the mycolic acid biosynthetic pathway via depletion of ketoymycolates and accumulation of hydroxymycolates, however, precise mechanism of its action is currently unclear. Baptista et al. (2018) proposed26) that mentioned compound might generate a toxic metabolite, methylglyoxal, which damaged the pathogen.
The PTM (3) molecule in a combination with BDQ (1) and linezolid (LNZ; 4) (Fig. 4) is approved for treating a limited and specific population of adult patients with XDR-TB, treatment-intolerant or non-responsive pulmonary MDR-TB27).
Due to the mechanisms of action that are different from those of other available anti-TB drugs, efficacy of the compounds (1–3) appeared optimal in cases of the adults with resistant pulmonary TB. Although drugs’ pharmacokinetic and pharmacodynamic profiles seem optimal as well, potential cardiovascular side effects of BDQ (1) and DLM (2), such as QT-interval prolongation, have been associated with their use. Moreover, there can be found also some “technical“ limitations of those modern anti-TB drugs, including high price and limited availability together with logistic issues in many regions of the world14, 17).
Following those obstacles, the development, preclinical and clinical investigations, approval and clinical use of highly effective and safe anti-TB agents to combat DR-TB, MDR-TB, or XDR-TB, is still major challenge and task. LNZ (4), sutezolid (STZ; 5), delpazolid (DPZ; 6), posizolid (PSZ; 7), or contezolid (CTZ; 8) are currently in various phases of clinical trials. These compounds (Figs. 4, 5 and 6), which inhibit protein synthesis due to 50S ribosomal subunit blockage of mycobacterial pathogens, contain a differently substituted oxazolidinone structural motif28).
In addition, the patient-centered approach with suitable case management strategies should be implemented in practice, taking into very strong consideration social, cultural and environmental aspects of the care. All-oral treatment regimens including new and repurposed drugs might be a preferable strategy for most patients. Injectable anti-TB agents should be avoided whenever possible6).
Development of a TB-vaccine also remains a critical global health priority given the deadly nature of ongoing TB-epidemic and spread of MDR-TB, or XDR-TB strains. Significant degree of protection against the TB disease shown by the M72/AS01E vaccine candidate, which is in a phase 2b clinical trial, strongly suggested that TB-vaccines are feasible and encouraging preclinical results from advanced vaccine candidates, such as a cytomegalovirus-vectored TB vaccine construct, offer the prospect of further progress29).
Perchlozone as a new armor in combating multidrug-resistant tuberculosis and extensively drug-resistant tuberculosis
Perchlozone (PCZ; 9), chemically 4-tioureidoiminomethylpyridinium perchlorate, was synthesized in 1990 in the A. E. Favorsky Irkutsk Institute of Chemistry (Siberian Branch of the Russian Academy of Sciences), founded in 1957 as one of the first academic institutes in the Eastern Siberia, in cooperation with St. Petersburg Research Institute of Phthisiopulmonology (Russian Federation). This compound (Fig. 7) belongs chemically into the class of thiosemicarbazones, whose anti-TB activity is well-known30–32).
X-Ray diffraction analysis of a crystal structure of PCZ (9) showed that both pyridine and thiosemicarbazone fragments were almost planar. The cation part of given molecule contained four hydrogen atoms attached to nitrogens (N1, N3 and N4) (Fig. 7), which were capable of hydrogen bonding. Relatively weak hydrogen bonds involving sulfur atoms of neighboring thiosemicarbazone chains were present and linked the cations into “dimers“33). The hydrogen bond was formed between N-H (N3 atom of first monomer) and S (second monomer) as well as N-H (N3 atom of second monomer) and S (first monomer).
The molecular anti-TB mechanism for thiosemicarbazones has not been understood clearly yet. Thiacetazone (TAZ; 10) (Fig. 8), also termed thioacetazone, tibione, or amithiozone, is an old, inexpensive anti-TB compound, which belongs structurally into the same “chemical“ class as PCZ (9). It was proposed that TAZ (10), as a prodrug, required activation by a mycobacterially encoded monoxygenase, EthA. The activated compound affected mycolic acid synthesis, probably by inhibiting cyclopropane mycolic acid synthases. In addition, thiosemicarbazone group chelated metal cations strongly and anti-TB efficiency of TAZ (10) was potentiated by copper23, 34, 35).
Therefore, anti-TB properties of PCZ (9) might be dependent on its conversion to one or more active agents. The EthA enzyme of M. tuberculosis would oxidize a “parent“ compound to a highly reactive sulfenic acid form, which might specifically covalently react with a cysteine residue of a hydroxyacyl-ACP-dehydratase (HadA) subunit of dehydratase (type II fatty acid synthase; FAS-II), thereby inhibiting formation of hydroxyacyl-ACP-dehydratase heterodimers (HadAB) of FAS-II36).
Moreover, a carbodiimide metabolite generated from a sulfenic acid intermediate via sulfenic acid form should react with mycothiol, a prinicipal thiol in mycobacteria, which protects the M. tuberculosis cell against oxidative damage and electrophilic toxins. Both sulfenic acid intermediate and carbodiimide metabolite might sensitize M. tuberculosis to oxidative damage37).
The PCZ (9) molecule showed bactericidal activity in vitro against M. tuberculosis Erdman, M. tuberculosis H37Rv, M. tuberculosis „Academia“, M. bovis bovinus 8 as well as 16 drug-resistant clinical isolates, including MDR-TB strains. Experiments employing animal models revealed therapeutic effect in vivo of PCZ (9) comparable to INH, AK and ofloxacin (OFX), respectively. In addition, PCZ (9) was even more efficient than EMB or ciprofloxacin (CPX) and was regarded as a more favorable therapeutic alternative than FQs. In summary, preclinical investigations of PCZ (9) confirmed its high activity in vivo against various TB and non-TB strains of mycobacteria as well as convenient pharmacokinetic and toxicological properties30).
Clinical phases of the research started at the State Research Center for Preventive Medicine (Moscow; current name is the National Research Center for Preventive Medicine) and the State Research Institute of Immunology, Russian Academy of Medical Sciences (Moscow) in 200938, 39).
Upon official approval of PCZ (9) in November 2012 and its introduction in routine clinical practice for the treatment of MDR-TB and XDR-TB, the effectiveness of short-term (6-months) therapies was significantly increased40, 41).
The use of concerned compound as an integral part of combination therapy regimens notably reduced the time of bacilli elimination in pulmonary TB caused by the DR-TB strains. It was observed that PCZ (9) gave patients suffering from the severest and epidemiogically poor form of TB a chance to recover42).
When PCZ (9) was included in a scheme for the treatment of HIV-1 negative patients, who were infected with M. avium, anti-inflammatory effect of the drug was observed42). Similarly, if BDQ (1) and PCZ (9) were introduced simultaneously as active components of very complex treatment regimens for patients suffering from MDR-TB, or XDR-TB, ceasing of mycobacterial excretion, closing of decay cavities, regression of inflammatory changes, elimination of the TB bacilli and achieving of positive X-ray dynamics, respectively, was observed43, 44).
Hypothyroidism is a well-known side effect of the MDR-TB treatment. However, only a few studies described an eventual relationship between the progression of this syndrome and anti-TB therapy based on PCZ (9). Results from a retrospective study involving the patients treated with PCZ (9) indicated that there was no need to replace given anti-TB drug with the other one(s) due to eventual worsening of hypothyroidism45).
The thiosemicarbazone scaffold-containing molecule (9), when being combined with antiretroviral therapy, was able to increase the number of CD4+ cells and concentration of a specific interleukin (IL-4), thus providing good prognostic value for the treatment of patients with HIV-1 and lung TB co-infection46). In addition, the inclusion of both BDQ (1) and PCZ (9) in complex chemotherapy of MDR-TB and XDR-TB in HIV-1 positive patients increased significantly the effectiveness of such treatment in terms of stopping mycobacterial excretion and closing decay cavities47).
Toxicity of PCZ (9) and its tolerability by the human organism were evaluated in relevant preclinical and clinical studies and all those parameters were acceptable, as published39). However, recent preliminary conclusions of a research team from the East-Siberian Institute of Medical and Ecological Research in Angarsk (Russian Federation), who aimed reactions in vivo of experimental (animal) models to subacute inhalation exposure of given drug, indicated compound’s genotoxicity48).
Besides, serious in vitro cytotoxicity issues were connected with other very promising anti-TB compounds, which contained a (substituted) thiosemicarbazone moiety31). Therefore, none of these substances was tested in vivo.
Nanoparticle-based drug formulations might be a promising way of how to avoid such toxicological obstacles. If experimental (animal) models suffering from MDR-TB were treated with intravenous immunoglobulin G (IgG)-modified nanoparticles and intraperitoneal IgG-modified microparticles, both loaded with PCZ (9), targeted particle delivery to the foci of infection in TB-animals was seen. Phagocytic macrophages of experimental animals internalized the particles and transported them to the foci of TB in inner organs. In addition, eventual toxicity of the drug was considerably reduced49).
Gopal and Dick (2015) found out that PCZ (9) and TAZ (10) affected a similar spectrum of mycobacterial strains and, in addition, might show a similar mechanism of action. The authors noted that possible cross-resistance of PCZ (9) and TAZ (10) with ethionamide (ETA) via EThA mutations should be kept in mind50).
The main limitation, which prevents more extensive use of PCZ (9) in the therapy of MDR-TB or XDR-TB, is that considered drug is practically not available outside the Russian Federation and has not been included in current recommendations of WHO, as published6, 7, 14).
Conclusions
The progress, which was achieved in the anti-TB compounds development together with consistent implementation of effective anti-TB programmes in the 21st century, formed preconditions for satisfactory management of TB in the world. However, it might be quite irresponsible to speak silently about a very close victory over the disease or even declare loudly the winning approaches. Following notable increase in the number of patients suffering from MDR-TB, or XDR-TB worldwide who could be, in addition, co-infected with HIV-1, the use of PCZ (9), as a very efficient and safe component of combination therapy regimens, improved markedly therapeutic and clinical outcomes of the treatment. It might be expected that PCZ (9) acted as a prodrug. Baeyer-Villiger monooxygenase (EthA) from Mycobacterium spp. has broad substrate specificity, being able to oxidize different compounds, including the ones containing a thiocarbamide, or thiosemicarbazone moiety. Thus, both highly reactive sulfenic acid intermediate and carbodiimide metabolite of PCZ (9) inhibit mycobacterial cell wall synthesis interfering with a dehydration step of the type II fatty acid synthase (FAS II) pathway and sensitize the M. tuberculosis cell to oxidative stress, respectively. Recent preliminary findings could indicate possible genotoxicity in vivo when experimental (animal) models were exposed to subacute inhalation of the drug. Therefore, it might be very reasonable to continue in more thorough and precise research focused on the selection of suitable formulations of PCZ (9). In this regard, nano- or microparticle-based drug formulations might be taken into strong consideration in order to eliminate (eventual) toxicity of this very powerful synthetic “weapon“ against MDR-TB and XDR-TB as well.
Acknowledgements
We would like to thank the Czech and Slovak Pharmacy Journal (Česká a slovenská farmacie) for the opportunity to publish the article.
Conflict of interest: none.
Assoc. Prof. PharmDr. Ivan Malík, PhD.1,2 (∗)
1Department of Pharmaceutical Chemistry, Faculty of Pharmacy,
Comenius University in Bratislava
Odbojárov 10, 832 32 Bratislava, Slovak Republic
2Institute of Chemistry, Clinical Biochemistry and Laboratory
Medicine, Faculty of Medicine, Slovak Medical University in
Bratislava, Limbová 12, 833 03 Bratislava, Slovak Republic
e-mail: malik@fpharm.uniba.sk
J. Čižmárik • M. Pecháčová
Department of Pharmaceutical Chemistry, Faculty of Pharmacy,
Comenius University in Bratislava, Slovak Republic
Sources
1. Vasava M. S., Bhoi M. N., Rathwa S. K., Borad M. A., Nair S. G., Patel H. D. Drug development against tuberculosis: Past, present and future. Indian J. Tuberc. 2017; 64, 252–275. doi: 10.1016/j.ijtb.2017.03.002
2. Hershkovitz I., Donoghue H. D., Minnikin D. E., May H., Lee O. Y., Feldman M., Galili E., Spigelman M., Rothschild B. M., Bar-Gal G. K. Tuberculosis origin: the Neolithic scenario. Tuberculosis 2015; 95(Suppl. 1), S122–S126. doi: 10.1016/j.tube.2015.02.021
3. World Health Organization (WHO). Global Tuberculosis Report 2019. Geneva: World Health Organization 2019; 297.
4. Chetty S., Ramesh M., Singh-Pillay A., Soliman M. E. S. Recent advancements in the development of anti-tuberculosis drugs. Bioorg. Med. Chem. Lett. 2017; 27, 370–386. doi: 10.1016/j.bmcl.2016.11.084
5. Koch A., Cox H., Mizrahi V. Drug-resistant tuberculosis: Challenges and opportunities for diagnosis and treatment. Curr. Opin. Pharmacol. 2018; 42, 7–15. doi: 10.1016/j.coph.2018.05.013
6. Mase S. R., Chorba T. Treatment of drug-resistant tuberculosis. Clin. Chest Med. 2019; 40, 775–795. doi: 10.1016/j.ccm.2019.08.002
7. Tiberi S., Zumla A., Battista Migliori G. Multidrug and extensively drug-resistant tuberculosis. Epidemiology, clinical features, management and treatment. Infect. Dis. Clin. North. Am. 2019; 33, 1063–1085. doi: 10.1016/j.idc.2019.09.002
8. Dadu M., Hovhannesyan A., Ahmedov S., van der Werf M. J., Dara M. Drug-resistant tuberculosis in Eastern Europe and Central Asia: a time-series analysis of routine surveillance data. Lancet Infect. Dis. 2020; 20, 250–258. doi: 10.1016/S1473-3099(19)30568-7
9. Belyaeva E., Chernokhaeva I., Sapozhnikova N., Nazarenko M., Starshinova A., Yablonskiy P. Factors predisposing to the development of extensive drug resistance of Mycobacterium tuberculosis. Medicinal Alliance (Medicinskiy Alyans; In Russian) 2017; 5, 51–56.
10. Ergeshov А. Е. Tuberculosis in the Russian Federation: Situation, challenges and perspectives. Annals of the Russian Academy of Medical Sciences (Vestnik RAMN; In Russian) 2018; 73, 330–337. doi: 10.15690/vramn1023
11. Meshkov I., Petrenko T., Keiser O., Estill J., Revyakina O., Felker I., Raviglione M. C., Krasnov V., Schartz Y. Variations in tuberculosis prevalence, Russian Federation: a multivariate approach. Bull. World Health Organ 2019; 97, 737–745. doi: 10.2471/BLT.19.229997
12. Yablonskii P. K., Vizel A. A., Galkin V. B., Shulgina M. V. Tuberculosis in Russia. Its history and its status today. Am. J. Respir. Crit. Care Med. 2015; 191, 372–376. doi: 10.1164/rccm.201305-0926OE
13. Augustynowicz-Kopeć E., Demkow U., Grzelewska--Rzymowska I., Korzeniewska-Koseła M., Langfort R., Michałowska-Mitczuk D., Rowińska-Zakrzewska E., Zielonka T. M., Ziołkowski J., Zwolska Z. Guidelines of Polish Respiratory Society concerning diagnosis, treatment and prevention of tuberculosis in adults and in children. Pneumol. Alergol. Pol. 2013; 81, 323–379.
14. Zellweger J.-P. Current options for the management of multi‑drug-resistant tuberculosis (review). Medicinal Alliance (Medicinskiy Alyans; In Russian) 2017; 6, 22–28.
15. Ryan N. J., Lo J. H. Delamanid: First global approval. Drugs 2014; 74, 1041–1045. doi: 10.1007/s40265-014-0241-5
16. Keam S. J. Pretomanid: First approval. Drugs 2019; 79, 1797–1803. doi: 10.1007/s40265-019-01207-9
17. Esposito S., Bianchini S., Blasi F. Bedaquiline and delamanid in tuberculosis. Expert Opin. Pharmacother. 2015; 16, 2319–2330. doi: 10.1517/14656566.2015.1080240
18. Sensi P. History of the development of rifampin. Rev. Infect. Dis. 1983; 5(Suppl. 3), S402–S406. doi: 10.1093/clinids/5.supplement_3.s402
19. Deltyba® (delamanid) approved in Russian Federation for treatment of pulmonary multidrug-resistant tuberculosis (MDR-TB). https://www.otsuka.co.jp/en/company/newsreleases/2020/ 20200525_1.html (20.9.2020)
20. Haagsma A. C., Podasca I., Koul A., Andries K., Guillemont J., Lill H., Bald D. Probing the interaction of the diarylquinoline TMC207 with its target mycobacterial ATP synthase. PLoS One 2011; 6, art. no. e23575 (7 pp.). doi: 10.1371/journal.pone.0023575
21. Hards K., Robson J. R., Berney M., Shaw L., Bald D., Koul A., Andries K., Cook G. M. Bactericidal mode of action of bedaquiline. J. Antimicrob. Chemother. 2015; 70, 2028–2037. doi: 10.1093/jac/dkv05
22. Sarathy J. P., Gruber G., Dick T. Re-understanding the mechanisms of action of the anti-mycobacterial drug bedaquiline. Antibiotics (Basel) 2019; 8, art. no. 261 (12 pp.). doi: 10.3390/antibiotics8040261
23. Alahari A., Trivelli X., Guérardel Y., Dover L. G., Besra G. S., Sacchettini J. C., Reynolds R. C., Coxon G. D., Kremer L. Thiacetazone, an antitubercular drug that inhibits cyclopropanation of cell wall mycolic acids in mycobacteria. PLoS One 2007; 2, art. no. e1343 (12 pp.). doi: 10.1371/journal.pone.0001343.
24. Xavier A. S., Lakshmanan M. Delamanid: A new armor in combating drug-resistant tuberculosis. J. Pharmacol. Pharmacother. 2014; 5, 222–224. doi: 10.4103/0976-500X.136121
25. Stover C. K., Warrener P., van Devanter D. R., Sherman D. R., Arain T. M., Langhorne M. H., Anderson S. W., Towell J. A., Yuan Y., McMurray D. N., Kreiswirth B. N., Barry C. E., Baker W. R. A small-molecule nitroimidazopyran drug candidate for the treatment of tuberculosis. Nature 2000; 405, 962–966. doi: 10.1038/35016103
26. Baptista R., Fazakerley D. M., Beckmann M., Baillie L., Mur L. A. J. Untargeted metabolomics reveals a new mode of action of pretomanid (PA-824). Sci. Rep. 2018; 8, art. no. 5084 (7 pp.). doi: 10.1038/s41598-018-23110-1
27. Conradie F., Diacon A. H., Ngubane N., Howell P., Everitt D., Crook A. M., Mendel C. M., Egizi E., Moreira J., Timm J., McHugh T. D., Wills G., Bateson A., Hunt R., van Niekerk Ch., Li M., Olugbosi M., Spigelman M., on behalf of Nix-TB Trial Team. Bedaquiline, pretomanid and linezolid for treatment of extensively drug resistant, intolerant or non-responsive multidrug resistant pulmonary tuberculosis. N. Engl. J. Med. 2020; 382, 893–902. doi: 10.1056/NEJMoa1901814
28. Reddy Tetali S., Kunapaeddi E., Prasad Mailavaram R., Singh V., Borah P., Deb P. K., Venugopala K. N., Hourani W., Tekade R. K. Current advances in the clinical development of anti-tubercular agents. Tuberculosis 2020; 125, art. no. 101989 (12 pp.). doi: 10.1016/j.tube.2020.101989
29. Schrager L. K., Vekemens J., Drager N., Lewinsohn D. M., Olesen O. F. The status of tuberculosis vaccine development. Lancet Infect. Dis. 2020; 20, 28–37. doi: 10.1016/S1473-3099(19)30625-5
30. Pavlova M., Vinogradova T., Starshinova A., Sapozhnikova N., Chernokhaeva I., Archakova L., Yablonskii P. Confirmation of preclinical studies data on thioureidoiminomethylpyridinium perchlorate (perchlozon) in clinic. Int. J. Curr. Res. 2015; 7, 14501–14505.
31. Opletalová V., Doležel J. Thiosemicarbazones and their antimycobacterial effects. Čes. slov. Farm. 2013; 62, 78–83.
32. Pavan F. R., da Silva Maia P. I., Leite S. R. A., Deflon V. M., Batista A. A., Sato D. N., Franzblau S. G., Leite C. Q. F. Thiosemicarbazones, semicarbazones, dithiocarbazates and hydrazide/hydrazones: anti-Mycobacterium tuberculosis activity and cytotoxicity. Eur. J. Med. Chem. 2010; 45, 1898–1905. doi: 10.1016/j.ejmech.2010.01.028
33. Smolentsev A. I., Lavrenova L. G., Elokhina V. N., Nakhmanovich A. S., Larina L. L. Crystal structure of pyridine-4-aldehyde thiosemicarbazone perchlorate and trifluoromethane sulfonate. J. Struct. Chem. 2009; 50, 500–504. doi: 10.1007/s10947-009-0076-1
34. Volynets G. P., Tukalo M. A., Bdzhola V. G., Derkach N. M., Gumeniuk M. I., Tarnavskiy S. S., Starosyla S. A., Yarmoluk S. M. Benzaldehyde thiosemicarbazone derivatives against replicating and nonreplicating Mycobacterium tuberculosis. J. Antibiot. (Tokyo) 2019; 72, 218–224. doi: 10.1038/s41429-019-0140-9
35. Dover L. G., Alahari A., Gratraud P., Gomes J. M., Bhowruth V. E. EthA, a common activator of thiocarbamide-containing drugs acting on different mycobacterial targets. Antimicrob. Agents Chemother. 2007; 51, 1055–1063. doi: 10.1128/AAC.01063-06
36. Grzegorzewicz A. E., Eynard N., Quémard A., North E. J., Margolis A., Lindenberger J. J., Jones V., Korduláková J., Brennan P. J., Lee R. E., Ronning D. R., McNeil M. R., Jackson M. Covalent modification of the Mycobacterium tuberculosis FAS-II dehydratase by Isoxyl and Thiacetazone. ACS Infect. Dis. 2015; 1, 91–97. doi: 10.1021/id500032q
37. Qian L., Ortiz de Montellano P. R. Oxidative activation of thiacetazone by the Mycobacterium tuberculosis flavin monooxygenase EtaA and human FMO1 and FMO3. Chem. Res. Toxicol. 2006; 19, 443–449. doi: 10.1021/tx050328b
38. Vinogradova T. I., Zabolotnykh N. V., Vasilyeva S. N., Vitovskaya M. L., Malygina E. I. Development of optimal schemes of drug-resistant tuberculosis therapy in experiment. Biology and Experimental Medicine (Biologia i Experimentalnyaya Medicina; In Russian) 2011; 28, 88–93.
39. Yablonskiy P. K., Vinogradova T. I., Levashev Y. N., Pavlova M. V., Zilber E. K., Starshinova A. A., Sapozhnikova N. V., Chernokhaeva I. V., Archakova L. I., Zabolotnykh N. V., Vitovskaya M. L. Preclinical and clinical trials of the new tuberculosis drug perchlozon. Therapeutic Archive (Terapevticheskii Arkhiv; In Russian) 2016; 88, 111–115. doi: 10.17116/terarkh2016883111-115
40. Chernokhaeva I. V., Pavlova M. V., Starshinova A. A., Belyaeva E. N., Sapozhnikova N. V., Gavrilov P. V., Zhuravlev V. Yu., Archakova L. I., Yablonskiy P. K. Efficacy of treatment by perchlozone of multidrug-resistant tuberculosis. Practical Medicine (Praktitscheskaya Medicina; In Russian) 2015; 1, 81–85.
41. Istomina E., Sokolovich E., Pavlova M., Archakova L., Sapozhnikova N., Chernokhaeva I., Yablonskiy P. Clinical example of effective treatment of the recurrence of tuberculosis with multiple dosing stability of mycobacteria. Medicinal Alliance (Medicinskiy Alyans; In Russian) 2019; 7, 57–64.
42. Starshinova A., Belyaeva E., Chernokhaeva I., Sapozhnikova N., Pavlova M., Nazarenko M., Esmedlyaeva D., Diyakova M., Yablonskiy P. Inflammatory response in patients with multiply drug resistant lung tuberculosis against the background of treatment including thioreidoiminomethylpyridinium perchlorate. Medicinal Alliance (Medicinskiy Alyans; In Russian) 2019; 7, 24–31.
43. Pavlova M. V., Ershova E. S., Sapozhnikova N. V., Starshi-nova А. А., Аrchakova L. I., Chernokhaeva I. V. A clinical case of effective treatment of extensive drug resistance tuberculosis with new anti-tuberculosis drugs. Tuberculosis and Lung Diseases (Klinitcheskoe Nablyudeni; In Russian) 2017; 95, 58–62, doi: 10.21292/2075-1230-2017-95-12-58-62
44. Pavlova M. V., Vinogradova T. I., Zabolotnykh N. V., Ershova E. S., Sapozhnikova N. V., Chernokhaeva I. V., Archakova L. I., Vitovskaya M. L., Dogonadze M. Z., Starshinova A. A., Grishko A. N., Yablonsky P. K. Prospects for the use of new generation of anti-tuberculosis drugs in treatment of drug-resistant tuberculosis (experimental and clinical study). Rev. Clin. Pharmacol. Drug Ther. 2018; 16, 33–40. doi: 10.17816/RCF16433-40
45. Potanina O. Hypothyroidism in tuberculosis patients receiving thioureidoiminomethylpyridinium perchlorate therapy. Medicinal Alliance (Medicinskiy Alyans; In Russian) 2018; 6, 41–45.
46. Strygin A. V., Klyausov A. S., Dotsenko A. M., Strygina A. O., Morkovin E. I. Effects of perchlozon and antibodies against human gamma-interferon and CD4 glycoprotein on the immune system of HIV-infected patients with associated pulmonary tuberculosis. Journal of VolgSMU (Vestnik VolgSMU; In Russian) 2017; 62, 42–46. doi: 10.19163/1994-9480-2017-2(62)-42-46
47. Pavlova M., Ershova E., Sapozhnikova N., Chernokhaeva I., Starshinova A., Anisimova A., Yablonskiy P. The first experience of bedaquiline and thioureidoiminomethylpyridinium perchlorate combination in patients with HIV infection. Medicinal Alliance (Medicinskiy Alyans; In Russian) 2019; 7, 22–29.
48. Sosedova L. M., Vokina V. A., Kapustina E. A., Bogomolova E. S. Evaluation of genotoxicity of perchlozone, antituberculous drug. Bull. Exp. Biol. Med. 2020; 169, 48–52. doi: 10.1007/s10517-020-04821-w
49. Churilov L., Korzhikov-Vlakh V., Sinitsyna E., Polyakov D., Darashkevich O., Poida M., Platonova G., Vinogradova T., Utekhin V., Zabolotnykh N., Zinserling V., Yablonsky P., Urtti A., Tennikova T. Enhanced delivery of 4-thioureidoiminomethylpyridinium perchlorate in tuberculosis models with IgG functionalized poly(lactic acid)-based particles. Pharmaceutics 2019; 11, art. no. 2 (20 pp.). doi: 10.3390/pharmaceutics11010002
50. Gopal P., Dick T. The new tuberculosis drug Perchlozone shows cross-resistance with thiacetazone. Int. J. Antimicrob. Agents. 2015; 45, 430–433. doi: 10.1016/j.ijantimicag.2014.12.026
Labels
Pharmacy Clinical pharmacologyArticle was published in
Czech and Slovak Pharmacy
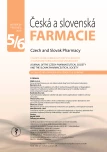
2020 Issue 5-6
Most read in this issue
- Meropenem serum concentrations in intensive care patients: a retrospective analysis
- K životnímu jubileu prof. RNDr. Jozefa Csölleiho, CSc.
- Development and validation of a HPLC method for quantifica-tion of degradation impurities of salbutamol sulfate with following long-term stability studies in multicomponent cough syrup
- Automated preparation of radiopharmaceuticals as a tool of radiation protection optimisation of staff