BIPHASIC CALCIUM PHOSPHATE SCAFFOLDS DERIVED FROM HYDROTHERMALLY SYNTHESIZED POWDERS
Autoři:
Ana S. Neto; José M. F. Ferreira
Působiště autorů:
Aveiro, Portugal
; Department of Materials and Ceramic Engineering, CICECO, University of Aveiro
Vyšlo v časopise:
Lékař a technika - Clinician and Technology No. 3, 2018, 48, 77-83
Kategorie:
Original research
Souhrn
Biphasic calcium phosphate (BCP) scaffolds were successfully produced by robocasting. The BCP powder was prepared by hydrothermal synthesis (150 °C for 4 h) and calcined at 1000 °C. The as-obtained powder was milled to obtain a suitable particle size distribution (PSD) for optimizing the rheological properties of the suspensions and pastes prepared thereof. Scaffolds with different pore dimensions (300×300, 500×500, 250×500 and 300×600 µm) were prepared by extruding the pastes through 410 µm diameter nozzles. The green scaffolds were dried and posteriorly sintered at 1100 °C. The compressive strength of the sintered scaffolds was well within the range of the mechanical properties reported from cancellous bone, being intrinsically related with the particle size distribution. Moreover, the obtained scaffolds demonstrated to have good biomineralization ability. The obtained scaffolds by robocasting revealed to possess promising features for their applications in bone regeneration and tissue engineering.
Keywords:
biphasic calcium phosphates, hydrothermal synthesis, robocasting
Zdroje
-
Oryan, A., Alidadi, S., Moshiri, A., Maffulli, N.: Bone regenera-tive medicine: classic options, novel strategies, and future directions. J. Orthop. Surg. Res., 2014, vol. 9, pp. 1–18.
-
Mouriño, V., Boccaccini, A. R.: Bone tissue engineering therapeutics: controlled drug delivery in three-dimensional scaffolds. J. R. Soc. Interface, 2010, vol. 7, no. 43, pp. 209–227.
-
Perez, R. A., Seo, S. J., Won, J. E., Lee, E. J., Jang, J. H., Knowles, J. C., Kim, H. W.: Therapeutically relevant aspects in bone repair and regeneration. Mater. Today, 2015, vol. 18, no. 10, pp. 573–589.
-
Lobo, S. E., Arinzeh, T. L.: Biphasic calcium phosphate ceramics for bone regeneration and tissue engineering applications. Materials, 2010, vol. 3, no. 2, pp. 815–826.
-
O’Brien, F. J.: Biomaterials & scaffolds for tissue engineering. Mater. Today, 2011, vol. 14, no. 3, pp. 88–95.
-
Bose, S., Vahabzadeh, S., Bandyopadhyay, A.: Bone tissue engineering using 3D printing. Mater. Today, 2013, vol. 16, no. 12, pp. 496–504.
-
Henkel, J., Woodruff, M. A., Epari, D. R., Steck, R., Glatt, V., Dickinson, I. C., Choong, P. F. M., Schuetz, M. A., Hutmacher, D. W.: Bone Regeneration Based on Tissue Engineering Conceptions — A 21st Century Perspective. Bone Res., 2013, vol. 1, no. 3, pp. 216–248.
-
Gupta, H. S., Guitia, F.: An effective morphology control of hydroxyapatite crystal via hydrothermal synthesis. Cryst. Growth Des., 2009, vol. 9, pp. 466–474.
-
International Standard ISO 23317:2014(E). Implants for surgery - In vitro evaluation for apatite-forming ability of implant materials. 2014.
-
Popa, A. C., Stan, G. E., Husanu, M. A., Mercioniu, I., Santos, L. F., Fernandes, H. R., Ferreira, J. M. F.: Bioglass implant-coating interactions in synthetic physiological fluids with varying degrees of biomimicry. Int. J. Nanomedicine., 2017, vol. 12, pp. 638–707.
-
Marques, C. F., Perera, F. H., Marote, A., Ferreira, S., Vieira, S. I., Olhero, S., Miranda, P., Ferreira, J. M. F.: Biphasic calcium phosphate scaffolds fabricated by direct write assembly: Mechanical, anti-microbial and osteoblastic properties. J. Eur. Ceram. Soc., 2017, vol. 37, no. 1, pp. 359–368.
-
Zou, F., Zhao, N., Fu, X., Diao, J., Ma, Y., Cao, X., Wan, S., Zhong, S., Wang, Y.: Enhanced osteogenic differentiation and biomineralization in mouse mesenchymal stromal cells on a β-TCP robocast scaffold modified with collagen nanofibers. RSC Adv., 2016, vol. 6, no. 28, pp. 23588–23598.
-
Houmard, M., Fu, Q., Saiz, E., Tomsia, A. P.: Sol-gel method to fabricate CaP scaffolds by robocasting for tissue engineering. J. Mater. Sci. Mater. Med., 2012, vol. 23, no. 4, pp. 921–930.
-
Miranda, M., Fernández, A., Saiz, E., Tomsia, A. P., Torrecillas, R.: Application of new forming and sintering techniques to obtain hydroxyapatite and β-TCP nanostructured composites. Int. J. Mater. Res., 2010, vol. 101, no. 1, pp. 117–121.
-
Sadat-Shojai, M., Khorasani, M. T., Dinpanah-Khoshdargi, E., Jamshidi, A.: Synthesis methods for nanosized hydroxyapatite with diverse structures. 2013, vol. 9, no. 8, pp. 7591–7621.
-
Sadat-Shojai, M., Atai, M., Nodehi, A.: Design of Experiments (DOE) for the Optimization of Hydrothermal Synthesis of Hydroxyapatite Nanoparticles. 2011, vol. 22, no. 3, pp. 571–582.
-
Sadat-Shojai, M., Khorasani, M.-T., Jamshidi, A.: Hydrothermal processing of hydroxyapatite nanoparticles—A Taguchi experimental design approach. J. Cryst. Growth. 2012, vol. 361, pp. 73–84.
-
Mohammadi, Z., Sheikh-Mehdi Mesgar, A., Rasouli-Disfani, F.: Preparation and characterization of single phase, biphasic and triphasic calcium phosphate whisker-like fibers by homogenous precipitation using urea. Ceram. Int, 2016, vol. 42, no. 6, pp. 6955–6961.
-
Lemos, A. F., Santos, J. D., Ferreira, J. M. F.: Influence of char-acteristics of starting hydroxyapatite powders and of deagglom-eration procedure, on rheological behaviour of HA suspensions. Mater. Sci. Forum, 2004, vol. 455–456, pp. 361–365.
-
Bose, S., Roy, M., Bandyopadhyay, A.: Recent advances in bone tissue engineering scaffolds. Trends Biotechnol., 2012, vol. 30, no. 10, pp. 546–554.
-
Tang, W., Lin, D., Yu, Y., Niu, H., Guo, H., Yuan, Y., Liu, C.: Bioinspired trimodal macro/micro/nano-porous scaffolds loading rhBMP-2 for complete regeneration of critical size bone defect. Acta Biomater., 2016, vol. 32, pp. 309–323.
-
Lee, H., Jang, T. S., Song, J., Kim, H. E., Do Jung, H.: The production of porous hydroxyapatite scaffolds with graded porosity by sequential freeze-casting. Materials, 2017, vol. 10, no. 4, pp. 1–12.
-
An, J., Teoh, J. E. M., Suntornnond, R., Chua, C. K.: Design and 3D Printing of Scaffolds and Tissues. Engineering, 2015, vol. 1, no. 2, pp. 261–268.
-
Sabree, I., Gough, J. E., Derby, B.: Mechanical properties of porous ceramic scaffolds: Influence of internal dimensions. Ceram. Int., 2015, vol. 41, no. 7, pp. 8425–8432.
-
Bose, S., Vahabzadeh, S., Bandyopadhyay, A.: Bone tissue engineering using 3D printing. Mater. Today, 2013, vol. 16, no. 12, pp. 496–504.
-
Carter, D. R., Schwab, G. H., Spengler, D. M.: Tensile fracture of cancellous bone. Acta Orthop. Scand., 1980, vol. 51, no. 1–6, pp. 733–741.
Štítky
BiomedicínaČlánek vyšel v časopise
Lékař a technika
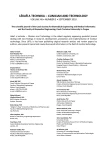
2018 Číslo 3
Nejčtenější v tomto čísle
- EEG MICROSTATES ANALYSIS IN PATIENTS WITH EPILEPSY
- EFFECT OF SAMPLING RATE ON THE ACCURACY OF MEASUREMENT OF NEONATAL OXYGEN SATURATION EXPOSURE
- BIPHASIC CALCIUM PHOSPHATE SCAFFOLDS DERIVED FROM HYDROTHERMALLY SYNTHESIZED POWDERS
- FLEXIBLE TEG ON THE ANKLE FOR MEASURING THE POWER GENERATED WHILE PERFORMING ACTIVITIES OF DAILY LIVING