Pulsed electric field energy calculation to damage red galangal (Alpinia purpurata, K. Scumm) rhizome slices and its essential oil yield and quality with hydrodistillation
Výpočet energie pulzního elektrického pole pro poškození plátků oddenku červeného galgánu (Alpinia purpurata, K. Scumm) a výtěžek a kvalita jeho silice při hydrodestilaci
Cílem této studie bylo stanovit množství energie, která poškodí buněčnou tkáň plátků oddenku červeného galgánu, a množství a kvalitu silice získaného destilací vodní parou. Studie měla randomizovaný blokový design, přičemž ošetření pulzním elektrickým polem (PEF) začínalo napětím 1000, 2000, 3000, 4000 a 5000 V a bez PEF a opakovalo se třikrát. Výsledky ukázaly, že napětí mezi 3000 a 4000 V (E = 120–160 V/cm) nebo ekvivalent 271,5–365,0 kJ/cm3 poškodilo buněčnou tkáň plátků oddenku červeného galgánu. Zvýšení výtěžnosti v důsledku PEF se pohybovalo od 13 % do 73 % a nedošlo ke změně hodnoty indexu lomu a specifické hmotnosti, ale došlo ke změně chemického složení složek silice. Přínosem výzkumu s ošetřením PEF je zvýšení výtěžku a zkrácení doby destilace.
Klíčová slova:
kvalita – oddenek galgánu – hydrodestilace – PEF – energie
Authors:
Sukardi Sukardi; Maimunah Hindun Pulungan; Sang Norma Lintang Asmara
Published in the journal:
Čes. slov. Farm., 2022; 71, 103-115
Category:
Původní práce
doi:
https://doi.org/https://doi.org/10.5817/CSF2022-3-103
Summary
This study aimed to determine the amount of energy to damage the red galangal rhizome sliced cell tissue and the amount and quality of the essential oil obtained by steam-water distillation. This study was a randomized block design, with pulsed electric field (PEF) voltage treatment starting at 1000, 2000, 3000, 4000, and 5000 V and without PEF and repeated three times. The results showed that the voltage between 3000 and 4000 V (E = 120–160 V/cm) or equivalent to 271.5–365.0 kJ/cm3 had damaged the red galangal rhizome slice cell tissue. The increase in yield due to PEF ranged from 13% to 73%, and there was no change in the value of the refractive index and specific gravity, but there was a change in the chemical composition of the essential oil constituents. The benefits of research with PEF treatment are the increase in yield and shorter distillation time.
Keywords:
galangal rhizome – hydrodistillation – quality – PEF – energy
Introduction
Pulsed electric field (PEF) as one of the emerging technologies works based on the potential transmembrane and does not cause damage to the existing chemical components because the increase in temperature is only 9–12 °C during exposure1). The potential transmembrane affects the ion gate channel on the cell membrane, and if it lasts for a long time, it affects the porosity. When a PEF device applies a voltage for a certain period to a material to damage cell tissue, the PEF requires specific input energy. Specific input energy requirements will increase when the time of application of PEF is also getting longer2). According to Walkling- Ribierio et al.3), as the electric field strength increases and the application time is longer, the requirement of specific input energy is greater which can cause more damage to the cell tissue. The increase in the applied electric field affects the decrease in the energy required to obtain the maximum level of damage4).
Cell electroporation is intended so that cells are damaged (broken/porous) without damaging the bioactive components5). Cells are surrounded by an insulating cell wall membrane made of cellulose, whereas the cytosol (cell contents) and extracellular fluid are electrolytes. At high frequencies, the cytosol, which is a macromolecule, initiates a characteristic relaxation process. Impedance occurs in the microwave range where the liquid dipoles show different dispersions so that the cells experience vibrations6).
The rupture of the cell membrane of the material occurs due to the energy supplied during the PEF pretreatment. PEF is an alternative technology with low heat energy, so it is called non-thermal pre-treatment1). In the process, the PEF uses 220–240 V and 300 W of power that allows the output voltage of the PEF device to increase to 10000 V so that energy can also be increased. The electrical energy generated from the PEF pre-treatment can be analyzed using a specific input energy calculation. The amount of specific input energy PEF depends on several things such as the anode/cathode distance, room dimensions, voltage (electric field strength), and treatment time7). The rupture of the cell wall will result in the liquid in the cell coming out and is called electroporation8). The application of PEF is very good for maintaining the product’s aromatic ingredients, because the heating treatment results in a loss of 22% of the aromatic material, whereas the PEF treatment loses only 3–9%. Other bioactive ingredients also had no effect due to PEF treatment9). The application of a PEF device in the industry not only is very efficient but also improves product quality10).
The extraction of plant bioactive compounds has been reported by many previous researchers using the solid/liquid extraction method. One of the factors that can positively affect the quality and quantity of the extraction results is the level of damage to the cell membrane. Physical, chemical, and biological treatments are used to accelerate the breakdown of cell membranes. PEF is considered a promising method for cellular tissue disruption without affecting the cell components10). The specific energy consumption (Q) of plant tissue damage using PEF treatment has been obtained in several studies, usually in the range of 1–15 kJ/kg11). Several researchers have previously reported that an amount of 6.4–16.2 kJ/kg is the energy consumption required for potato slice cell tissue12), 2.5 kJ/kg to damage red beet tissue13), 3.9 kJ/kg to damage sugar beet tissue14), and 10 kJ/kg to damage chicory root tissue15). The effect of low energy PEF (3–10 J/kg) on the extraction of polyphenols from grape seed has been reported16). Specific energy input (Q) has been identified as the main parameter of PEF pre-treatment before the extraction process. Treatment using 32 kJ/kg specific energy for apple juice extraction has been found to increase yield from 71.1% to 76.3%17). The specific energy input required for the extraction of the algae suspension (100 g dry weight per kg) is 1 MJ/kg18). An increase in the electric field strength (E) results in a decrease in the energy required to induce maximum plant cell tissue damage. The amount of energy received by the material needs to be calculated because it is related to the level of damage and the porosity of the cell wall. The increase in the number of porous cell walls increased the amount of extract obtained.
The application of PEF to determine the integrity of onion cell membranes has been reported by Ersus and Barrett19), beet tissue cell membrane integrity by Kulshrestha and Sastry20), apple fruit network by Chalermchat et al.21) and Bousetta et al.22), and alfalfa network by Gachovska et al.4). The application of PEF before extraction has been widely performed including the extraction of plant bioactive ingredients by Wijngaard et al.23), orange peel by Lungeo et al.24), red beet by Loginova et al.25), mushroom and celery leaf by Kusnadi and Sastry26), the root of the chicory plant by Loginova et al.15), chlorophyll extraction of spinach and tomato leaves by Aktas and Yildiz27), apples by Rawson et al.28) and Turk et al.17), and microalgae by Goettel et al.18). All of them show a positive influence on the results and the quality of the products obtained. Information to damage red galangal rhizome cell tissue with PEF has not been found so far. Therefore, this research is essential in science about PEF in tubers, especially galangal rhizomes.
This study aimed to improve the quality and quantity of galangal rhizome essential oil products obtained before distillation, with the finding of the minimum amount of energy required to damage cell membranes29). The PEF pre-treatment is expected to reduce energy consumption in the refinery so that production costs to obtain essential oils can be reduced.
Experimental part
Material preparation
Fresh red galangal rhizome was collected from a farmer’s garden in Tumpang-Malang Regency, Indonesia with 80 ± 5% water content. Red galangal rhizomes aged 12 months harvested were cleaned of dirt and soil by washing with water and drained (Fig. 1a). Clean rhizomes were sliced 0.5 ± 0.01 cm thick (Fig. 1b).
PEF treatment
The PEF generator is a local product but has been calibrated in a high-voltage laboratory, Faculty of Engineering, Universitas Brawijaya. The PEF generator requires 300 W of electrical power, capable of operating at a voltage of 5 V – 15 kV, frequency 5–15 kHz, and exposure time 5 s – 2 h. The cathode–anode distance can be adjusted from 5 to 40 cm and is made of SS 316 iron with a thickness of 1 mm. The chamber is made of acrylic with a diameter of 12 cm and a thickness of 0.2 mm. The schematic of the PEF tool is presented in Figure 2.
The PEF generator generates an electric field (AC or DC), and the voltage is regulated via a button and detected by a multimeter. The frequency and time of exposure are also set with the desired adjustment knob. Then, the material is placed into the chamber (between the cathode and anode), and the PEF generator is turned on and waited for the specified time. An electric field occurs between the cathode and the anode so that damage/porosity of the cell wall occurs. Furthermore, the material is taken from the chamber and followed by distillation to obtain the essential oil. In this research, DC current was used for the experiment and it carried out as follows: weighed 3000 g of sliced red galangal rhizome in a plastic bag container and then treated with a voltage of 0, 1000, 2000, 3000, 4000, and 5000 V (at a frequency of 5000 Hz, exposure time of 30 min, and cathode/anode distance of 25 cm). Each treatment was repeated three times. It takes 30 min of exposure and 5000 Hz frequency, because based on previous research for materials in the form of sliced rhizomes, the oil results obtained less than 30 min are not optimal.
Microscopic analysis (SEM)
The shape changes of cells in the galangal rhizome slice were observed before and after PEF exposure. Microscopic observations were performed on dry rhizome using a scanning electron microscope (SEM; FEI-Inspect S25-EDAX). Fresh galangal rhizome slices are dried in an oven at 50 °C during 2 × 24 h and transferred to the laboratory. The dry galangal rhizomes were cut 1 × 1 cm and then performed using sputter coating with gold coated SC-2620 (Emitech, France). Other galangal slices after PEF with 3000, 4000, and 5000 V were dried in an oven at 50 °C during 2 × 24 h, transported to the laboratory, and then cut 1 × 1 cm for coating with SC-2620 gold (Emitech). Sample were subsequently observed above.
Input energy calculation
The specific input energy of PEF in kJ/cm3 (WPEF) is calculated using Equation [1]. It is calculated by multiplication of voltage in V (U), total duration of PEF (t), the resistance of electrode (Rc), and volume chamber (Vc)29).
WPEF = U x t x Rc−1 x Vc−1 [1]
Distillation
Steam-water distillation was performed for 6 h at a temperature of 120 °C and at a pressure of 1.1 bar, and the amount and quality of the galangal essential oil obtained was calculated.
Gas chromatography – Mass spectrometry (GC-MS) analysis
Tests were conducted to determine the phytochemical content of essential oils produced using gas chromatography/mass spectrometry (GC-MS). The analysis was performed by injecting the sample into the instrument with the injection port conditions up to 250 °C, oven temperature up to 60 °C, and increasing periodically to 280 °C. The detector used is a MS with ionization energy of 70 eV, scan mass range (m/z) 28–600, with a detector temperature of 250 °C. The spectrum obtained was matched to the supporting literature. Further identification is carried out by comparing the mass spectra of the tested sample components with a database stored on a computer.
Yield calculation
Samples of raw materials were weighed using an analytical balance and recorded as the weight of the starting material. The results of the final treatment were weighed using an analytical balance and recorded as the weight of the resulting product. The yield of the material can be calculated using the formula as stated in Equation [2]30): %
Density analysis
Density measurements in essential oils are ideally carried out at a room temperature of ± 20 °C. The following is the procedure for measuring the density of essential oils using a pycnometer based on SNI30) Equation [3]:
1. Weigh the pycnometer empty and clean.
2. Fill the pycnometer with distilled water then cover it and weigh it.
3. Empty the pycnometer and clean with ethanol.
4. Fill the pycnometer with oil then cover it and weigh it.
5. Determine the density of the essential oil using the following formula:
Density = (m2-m)/(m1-m) [3]
with:
m – mass pycnometer empty (g),
m1 – mass pycnometer and aquades (g),
m2 – mass pycnometer and essential oil (g).
Refractive index analysis
The determination of the refractive index of essential oils is ideally carried out at a room temperature of 20 °C. The following is the procedure for measuring the density of essential oils using a refractometer30):
1. Pour alcohol on the surface of the refractometer prism and wipe with a tissue to make it sterile and clean.
2. Drop the essential oil whose refractive index will be measured on the refractometer prism.
3 Let it stand for a while so that the temperature of the oil and the tool is stable.
4. Read the numbers that appear on the refractometer layer.
Results and discussion
Microstructure of galangal rhizome
The galangal rhizome slices after being treated with PEF were dried in an oven at 50 °C for 2 × 24 h and then analyzed by SEM. SEM test results of slices of galangal rhizome without and with PEF 3000, 4000, and 5000 V are presented in Figure 3 (a, b, c, d). SEM is a type of electron microscope that can be used to identify the shape of nanoparticles of a material31). Based on the results of SEM micrographs, samples without PEF have dense cavities and tend to be closed. At 3000 V voltage treatment, the cell cavity began to open and opened maximally at 4000 V voltage treatment. The cell cavity began to be partially closed when the 5000 V treatment was applied, this was because the cell began to experience damage due to high-voltage application. The electric voltage applied to the material can damage the cell wall due to the electric current31, 32). The presence of larger cavities in the PEF-treated material is due to electroporation and joining with each other33).
The PEF treatment between 3000 and 4000 V has reached stage-2 creation of small metastable hydrophilic pores when the transmembrane potential has increased and stage-3 evolution of the pore population changes in pore number and/or size34). During the application of electric pulses to the spherical cell, elastic deformation of the membrane occurs that affects the induced transmembrane potential, the dynamics of the pore formation, and the maximum membrane deformation, also maximum pore opening35).
Plant plasma membranes are more affected with longer pulses than with short pulses36). Plant cell walls are composed of cellulose and other materials such as lignin37). The cell wall experiences stress and changes in the pores when given a PEF depends on the electric field strength, frequency, and exposure time23). The increase in voltage results in the occurrence of gate ion channel on the cell wall increasing to form pores38).
Energy calculation
Specific energy input is required to damage the cell wall or tissue before the extraction process is performed. There are several techniques to calculate the specific energy input to the material, including using the formula for the minimum energy required to damage the material per unit weight of the sample39). Buckow et al.40) stated that the energy required to solve the sample can be calculated by the specific energy (Qspec). The energy of the PEF treatment is calculated by Equation [1]29). The data show that increasing specific energy input is proportional to the increase in PEF voltage (Table 1).
Based on the calculation of the specific energy input (WPEF) and the results of the SEM analysis of the galangal rhizome slices (Figure 3), it can be seen that the tissue damage appears to be massive starting at a voltage between 3000 and 4000 V or equivalent to 271.5–362.0 kJ/cm3. The PEF exposure time is 30 min because the material is wet, so the water affects the electric field in the chamber. The amount of specific energy input is based on the energy stored in the capacitor and is influenced by voltage, capacitor storage capacity, frequency, and amount of material1). The amount of energy received by the material during PEF treatment is influenced by peak stress, total time, resistance, and chamber volume29). The minimum energy required to damage cell tissue is determined by voltage, current strength, number of pulses, duration of modality, exposure time, and material flow velocity17).
The electric voltage applied to the cell causes pores to form in the cell wall41). The increase in stress applied to the material is followed by an increase in the specific energy of the input received42) so that the gap damage is more massive and the material is easily extracted. Furthermore, it is said that the energy per pulse of the electric circuit element at time t1 is the power integral for each time. The effectiveness of treatment with PEF technology depends on the intensity of the electric field strength and the total energy applied and the characteristics of biological cells such as morphology, electrical conductivity, and chemical composition43).
The increasing electric field strength and the length of time of the application of PEF are followed by the greater the specific input energy required, resulting in more cell damage3). The increase in the applied electric field affects the decrease in the energy required to obtain the maximum level of damage4). The greater the specific energy input, the greater the exposure time and the PEF electric field strength.
The higher the applied voltage followed the higher the electric field strength produced, and the processing time can be reduced2). Extraction of rose oil obtained an increase of 46% and reduced distillation time with optimal input energy of 10 kJ/kg material44). The PEF with an energy of 100 kJ/kg and electric field strength (E) of 5 kV/cm on Arabidopsis thaliana cell walls has experienced porosity45). Experimental study of the effect of low energy pulsed electric charges (3–10 J/kg) on the extraction of grape seed polyphenols has been carried out by Boussetta et al.46). The electric field strength shows a threshold effect, whereas the specific energy input can be applied as a dose parameter. It depends on the type of application and the specific energy input required, and the permeabilization of plant tissue is in the range of 5–10 kJ/kg47). The energy requirement for pressing potato slices is 7.5 kJ/kg materials, and the application of PEF before pressing potato slices is more effective than that of pressing without PEF48). The amount of specific energy input to damage the cell depends on the cathode–anode distance and the dimensions of the chamber40). Damage to the apple slice tissue requires an electric field of 900 V/cm, and the damage to the apple slice tissue is irreversible21).
Essential oil yield and quality
Damage to the membrane minimizes the energy required to remove the material from the cell so that extraction is efficient, which in turn can increase extraction and obtain large amounts of oil49). Red galangal essential oil is an essential oil that can be obtained from the distillation of red galangal flowers, stems, and leaves50). According to Damayanti et al.51), the demand for red galangal essential oil in the market is very high, because it can be used in aromatherapy.
The compounds in the essential oil of red galangal (Alpinia purpurata, K. Schum) consist of 1,8-cineole (40.92%), acetyl chavicol (10.33%), cis β-farnesene (6.91%), l-cayophilene (6.32%), 1-β-bisabolene (3.37%), β-elemene (3.23%), α-pinene (3.20%), β-sesquiphellandrene (2.32%), β-pinene (2.21%), germacrene D (1.90%)52), and the rest d-pinene, galangin, and eugenol as the cause of the spicy taste of galangal53). Cineol (1,8-cineole) is a naturally occurring monoterpene, also known as eucalyptol. This compound is one of the main compounds in many plant essential oils. Cineol has been shown to have therapeutic benefits in inflammatory airway diseases, such as asthma and chronic obstructive pulmonary disease54).
Table 2 shows that the average yield of red galangal essential oil ranges from 0.30% to 0.52%. The highest yield was obtained at a 4000 V voltage treatment of 0.52%. The lowest yield was obtained at a 1000 V voltage treatment of 0.30%, this is similar to the research of Yajun et al.55). This event is due to the potential difference between the inside and the outside of the cell membrane that becomes larger due to electrostatic forces disintegrating organelles and cellular structures and an increase in the release of volatile substances in the material56). The intensity of the stress applied to the material that is too large will harm the distillation results, one of which is a decrease in yield57). The application of the PEF method as a pre-treatment in the extraction process is very good for the industry11).
Refractive index measurements were carried out to identify the purity of the oil58). The higher the refractive index, the better the oil quality59). There was no significant difference between the value of the refractive index and specific gravity between no PEF and PEF treatment; however, there was a slight increase in PEF treatment (Table 2). The more long-chain components such as sesquiterpenes or functional groups that contain oxygen, the more difficult the incoming light will be to refract60). Pre-treatment of PEF with higher voltage shows a decrease in the refractive index61). Pre-treatment of PEF with higher voltage can cause the pores of the cell membrane to open so that the oil is extracted more optimally and produces a higher refractive index62).
The density of essential oils is one of the benchmarks that can be used as a measure of quality63). According to Ospina et al.64), the density of essential oils is related to the composition of compounds contained in the oil itself. The average density of red galangal essential oil ranged from 0.8682 to 0.8879 g/ml. The highest density was obtained at a 4000 V treatment of 0.8879 g/ml. The lowest density was obtained in the control treatment of 0.8682 g/ml (Table 2).
Pre-treatment of PEF with higher voltage can cause the pores of the cell membrane to open so that the oil is extracted more optimally and produces a higher refractive index62). However, in this study, it showed a decrease in the refractive index at 5000 V treatment that could be due to the cell membrane having been severely damaged so that volatile compounds were lost and produced oil and the density was smaller than 4000 V. The results of the test with GC-MS obtained several chemical components in the distillation of galangal oil without PEF (control), PEF 1000 V, PEF 2000 V, PEF 3000 V, PEF 4000 V, and PEF 5000 V and based on 20 chemical components that have an average % area above 1%, as presented in Table 3.
GC-MS analysis
The application of PEF to biological cells resulted in electroporation of the cell wall membrane and increased cell wall permeability and facilitated the release of intracellular compounds65). The degree of disintegration of the cell wall appears to be highly dependent on the PEF exposure time and the electric field strength (E). At long PEF exposure times, a smaller electric field is required, as is the effect of pulse duration on PEF efficiency in sugar beet11). The change of the chemical component of essential oil with PEF treatment of the galangal rhizome is presented in Table 3.
The percentage of the macro component of patchouli oil decreased, but the micro component increased, this indicates an increase in quantity due to PEF treatment in patchouli oil extraction. Changes in the chemical components of patchouli essential oil also occurred due to the electrochemical reaction, namely isomerization, resulting in a decrease in macro components and an increase in micro components66). Judging from the composition of volatile compounds, PEF is a technology to improve the quality of aromatic compounds7). Boussetta et al.46) stated that the PEF treatment is influenced by several operating parameters such as the duration and strength of the electric field. The results of Garde- Cerdan et al.67) showed that the effect of applying PEF differed depending on the plant variety, but the aromatic profile of the studied material was not affected in any way at low electric fields. PEF increases antioxidant activity due to the formation of derivative compounds68).
Pataro et al.69) stated that the PEF pre-treatment of tomato peels at a field strength of 0.5–5 kV/cm (specific energy input 0.5–20 kJ/kg) reveals that lycopene is the main carotenoid extracted and there is no degradation/ isomerization phenomenon. Isomerization is a process of changing the configuration of atoms or groups of atoms in space. In complex compounds, isomerism occurs in complexes with a structure of two substituents or two kinds of ligands. Substituents can be located next to or opposite each other. If the substituent groups are located next to each other, then the isomer is a Cis isomer; otherwise, if the substituents are opposite each other, the isomer that occurs is a Trans isomer. PEF affects the VIT-C conformation, which induces the VIT-C isomer to change the enol form to the keto form. In addition, PEF treatment did not damage VIT-C70).
The application of a pulsed electric field (PEF) at high voltages tended to change the amount of several components of the volatile oil compounds (Table 3). Changes in the chemical components of red galangal rhizome oil due to the application of PEF occurred at the application of energy above 90 kJ/cm3. Previous researchers showed that the application of PEF with an energy of 20 kJ/kg on herbal plants resulted in the instability of the material so it required lower energy. The application of 10 kJ/kg energy obtained aroma and chemical composition of essential oils better than 20 kJ/kg71). The application of PEF with an energy of 10 kJ/kg and 20 kJ/kg and a distillation time of 0.5 h to 2.5 h, also resulted in changes in the yield and chemical components of rose flower oil72).
In addition, the application of PEF to essential oils will influence the quality of the oil. This is an important criterion since the atomic chains C14 to C24 are sensitive to energy above 10 kJ/kg72). The change in eucalyptol composition was affected by the application of high voltages. The quality of essential oils followed the trend of changing yields. At the energy input of 10 kJ/kg and 20 kJ/kg, the amount of distilled components according to their volatility also differs66). The essential oils obtained from the PEF treatment with energy less than 10 kJ/kg tended to have the same trend in the values of the chemical components73). The investigated compound of A. purpurata rhizome oil showed a significant difference (Table 3) at the application of energy above 90 kJ/cm3. Despite the differences in the structure of the chemical profile of A. purpurata, the changes in chemical components of A. purpurata oil had the same tendency to increase. It was consistent with the application of PEF to roses (Rosa alba L.)73).
The main components in essential oils are monoterpenes that act as taste and odor givers where monoterpenoids, sesquiterpenoids, diterpenoids, triterpenoids, tetraterpenoids, and polyterpenoids are part of terpenoid compounds (one of the secondary metabolites). The characteristics of monoterpenes and sesquiterpenes are volatile, diterpene compounds are less volatile, and triterpenes are non-volatile74). The main compounds contained in each treatment have differences. Eucalyptol, chavicol, α-terpineol, terpinene-4-ol, and β-pinene compounds are monoterpenes. Meanwhile, β-farnesene, α-cadinol, caryophyllene, and β-copaene compounds are classified as sesquiterpene compounds.
Based on Table 3, there are five main compound components contained in red galangal essential oil without PEF pre-treatment: eucalyptol 27.35%, β-farnesene 11.64%, β-pinene 8.70%, chavicol 6.40%, and terpinene-4-ol 4.31%. Treatment of PEF 1000 V contained five main compounds: eucalyptol 27.35%, β-myrcene 11.64%, β-pinene 8.70%, β-farnesene and phenol, 4-(2-propenyl) 6.40%, and phenol, 4-(2-propenyl)- acetate 4.31%. The 2000 V treatment contained five main compound components: eucalyptol 15.86%, alloaromadendrene 7.40%, phenol, 4-(2-propenyl)-, acetate 6.62%, β-pinene 5.86%, and α-cadinol 5.76%. At the 3000 V treatment, there are five main compounds: eucalyptol 29.03%, α-terpineol 14.18%, terpinene-4-ol 11.12%, chavicol 8.88%, and β-pinene 7.89%. At the 4000 V treatment, there were five main compounds: chavicol 9.04%, eucalyptol 8.99%, β-farnesene 8.67%, α-cadinol 7.85%, and β-copaene 6.52%. In the 5000 V treatment, there were five main compounds: eucalyptol 12.21%, chavicol 9.84%, β-farnesene 6.71%, α-cadinol 6.68%, and caryophyllene 6.01%. GC-MS analysis of A. purpurata essential oil revealed the presence of 42 essential oil components with -pinene, and -caryophyllene being the main constituents75). The A. purpurata extracted by chloroform contained an unstable lambda diterpene, lambda-817), 12-diene-15, 16-dial, and alkaloid piperine76). Based on the results, the application of PEF at 4000 V or 362 kJ/cm3 energy could change the volatile compound of essential oil. It decreased the yields due to the evaporation of the volatile compounds during distillation. The decreased volatile compound in A. purpurata extract could be avoided by applying PEF in the energy range of 100–200 kJ/cm3. The quality of A. purpurata essential oil was determined by volatile compounds such as terpene alcohol (terpineol) which determine the aromatic properties of essential oil. There is a wealth of scientific evidence supporting the wide application of galangal in food and its medicinal properties, such as antiviral, cardiovascular, and neuroprotective properties, along with preclinical and clinical studies with galangal bioactive compounds77).
Conclusion
The conclusion that can be conveyed in this study is that the PEF treatment of red galangal rhizome slices can damage cell tissue at a voltage between 3000 and 4000 V or equivalent to 271.5–365.0 kJ/cm3. Essential oil yield increased between 13% and 73% compared to no PEF treatment, so it is very prospective to be developed. There was no significant change in the density and refractive index of essential oils produced without PEF compared to PEF treatment. There was a decrease in the amount of some chemical components that make up essential oils, but most of them increased in the number of components and even formed or there were new components that were also distilled by PEF treatment. In the future, the application of PEF is very good for obtaining high yields; however, it is necessary to find the optimum conditions so that no changes in chemical components occur.
Acknowledgment
The project was supported by the Faculty of Agriculture Technology, Universitas Brawijaya. We would like to thank the Dean for the facilities given for the realization of this work.
Conflict of interest: none.
Received February 1, 2022 / Accepted April 4, 2022
Sukardi Sukardi, Maimunah Hindun Pulungan, Sang Norma Lintang Asmara
Department of Agro-industrial Technology
Faculty of Agriculture Technology, Brawijaya University, Malang, Indonesia
Jalan Veteran
Malang 65145, East Java, Indonesia
e-mail: sukardi@ub.ac.id
Zdroje
1. Töpfl S. Pulsed electric fields (PEF) for permeability of cell membranes in food and bioprocessing applications, process and equipment design and cost analysis. 2006 https://depositonce.tu-berlin.de/bitstream/ 11303/1738/1/Dokument_49.pdf/
2. Putri R. I., Syamsiana I. N., Hawa L. C and Meilany D. Aplikasi mikrokontroller pada pembangkit pulsa tegangan tinggi dengan pengaturan waktu pengolahan untuk pasteurisasi sari buah apel [Application of microcontrollers in high voltage pulse generators with processing time settings for pasteurization of apple juice]. INKOM Journal 2009; 3, 31–40.
3. Walkling-Ribeiro M., Noci F., Cronin D. A., Riener J., Lyng J. G., Morgan D. J. Reduction of Staphylococcus aureus and quality changes in apple juice processed by ultraviolet irradiation, pre-heating and pulsed electric fields. J. Food Eng. 2008; 89, 267–273.
4. Gachovska T. K., Adedeji A. A., Ngadi M. O. Influence of pulsed electric field energy on the damage degree in alfalfa tissue J. Food Eng. 2009; 95, 558–563.
5. Sack M., Sigler J., Frenzel S., Eing C., Arnold J., Michelberger T., Frey W., Attmann F., Stukenbrock L., Müller G. Research on industrial-scale electroporation devices fostering the extraction of substances from biological tissue. Food Eng. Rev. 2010; 2, 147–156.
6. Pliquett U. Bioimpedance: A review for food processing. Food Eng. Rev. 2010; 2, 74–94.
7. Sukardi S. Electroporation of Cell Membranes to Increase the Efficiency of Patchouli Oil Distillation (Pogostemon cablin Benth). Dissertation, Universitas Brawijaya, Malang 2016.
8. Jiahui L., Xinlao W., Yonghong W., Gongqiang L. Analysis for relationship of transmembrane potential–pulsed electric field frequency. Food Bioprod. Process 2009; 87, 261–265.
9. Soliva-Fortuny R., Balasa A., Knorr D., Martín-Belloso O. Effects of pulsed electric fields on bioactive compounds in foods: A review. Trends Food Sci. Technol. 2009; 20, 544–556.
10. Donsì F., Ferrari G., Pataro G. Applications 20 of pulsed electric field treatments for the enhancement of mass transfer from vegetable tissue. Food Eng. Rev. 2010; 2, 109–130.
11. Vorobiev E., Lebovka N. Pulsed-electric-fields-induced effects in plant tissues: Fundamental aspects and perspectives of applications. In: Vorobiev E., Lebovka N. (Edits) Electrotechnologies for Extraction from Food Plants and Biomaterials. New York: Springer 2009; 39–81.
12. Angersbach A., Knorr D. High intensity electric field pulses as pretreatment for effecting dehydration characteristics and rehydration properties of potato cubes. Nahrung (Germany) 1997; 41, 194–200.
13. López N., Puértolas E., Condón S., Raso J., Álvarez I. Enhancement of the solid-liquid extraction of sucrose from sugar beet (Beta vulgaris) by pulsed electric fields. LWT – Food Sci. Technol. 2009; 42, 1674–1680.
14. López N., Puértolas E., Condón S., Raso J., Alvarez I. Enhancement of the extraction of betanine from red beetroot by pulsed electric fields. J. Food Eng. 2009; 90, 60–66.
15. Loginova K. V., Shynkaryk M. V., Lebovka N. I., Vorobiev E. Acceleration of soluble matter extraction from chicory with pulsed electric fields. J. Food Eng. 2010; 96, 374–379.
16. Zderic A., Zondervan E., Meuldijk J. Breakage of cellular tissue by pulsed electric field: Extraction of polyphenols from fresh tea leaves. Chem. Eng. Trans. 2013; 32, 1795–1800.
17. Turk M. F., Vorobiev E., Baron A. Improving apple juice expression and quality by pulsed electric field on an industrial scale. LWT – Sci. Technol. 2012; 49, 245–250.
18. Goettel M., Eing C., Gusbeth C., Straessner R., Frey W. Pulsed electric field assisted extraction of intracellular valuables from microalgae. Algal Res. 2013; 2, 401–408.
19. Ersus S., Barrett D. M. Determination of membrane integrity in onion tissues treated by pulsed electric fields: Use of microscopic images and ion leakage measurements. Innov. Food Sci. Emerg. Technol. 2010, 11, 598–603.
20. Kulshrestha S. A., Sastry S. K. Changes in permeability of moderate electric field (MEF) treated vegetable tissue over time. Innov. Food Sci. Emerg. Technol. 2010; 11, 78– 83.
21. Chalermchat Y., Malangone L., Dejmek P. Electropermeabilization of apple tissue: Effect of cell size, cell size distribution and cell orientation. Biosyst. Eng. 2010; 105, 357–366.
22. Boussetta N., Lesaint O., Vorobiev E. A study of mechanisms involved during the extraction of polyphenols from grape seeds by pulsed electrical discharges. Innov. Food Sci. Emerg. Technol. 2013; 19, 124–132.
23. Wijngaard H., Hossain M. B., Rai D. K., Brunton N. Techniques to extract bioactive compounds from food by-products of plant origin. Food Res. Int. 2012; 46, 505– 513.
24. Luengo E., Álvarez I., Raso J. Improving the pressing extraction of polyphenols of orange peel by pulsed electric fields. Innov. Food Sci. Emerg. Technol. 2013; 17, 79–84.
25. Loginova K. V., Lebovka N. I., Vorobiev E. Pulsed electric field assisted aqueous extraction of colorants from red beet. J. Food Eng. 2011; 106, 127–133.
26. Kusnadi C., Sastry S. K. Effect of moderate electric fields on salt diffusion into vegetable tissue. J. Food Eng. 2012; 110, 329–336.
27. Aktas E. T., Yildiz H. Effects of electroplasmolysis treatment on chlorophyll and carotenoid extraction yield from spinach and tomato. J. Food Eng. 2011; 106, 339– 346.
28. Rawson A., Patras A., Tiwari B. K., Noci F., Koutchma T., Brunton N. Effect of thermal and non thermal processing technologies on the bioactive content of exotic fruits and their products: Review of recent advances. Food Res. Int. 2011; 44, 1875–1887.
29. Zhang Q., Barbosa-Cánovas G. V., Swanson B. G. Engineering aspects of pulsed electric field pasteurization. J. Food Eng. 1995; 25, 261–281.
30. SNI, SNI Minyak kayu putih [SNI Eucalyptus oil]. 2014. https://pesta.bsn.go.id/produk/detail/10197 -sni39542014
31. Jelin F. J., Kumar S. S., Malini M., Vanaja M., Annadurai G. Environment-assisted green approach AgNPs by nutmeg (Myristica fragrans): Inhibition potential accustomed to pharmaceuticals. Eur. J. Biomed. Pharm. Sci. 2015; 2, 258–274.
32. Janositz A., Knorr D. Microscopic visualization of pulsed electric field induced changes on plant cellular level. Innov. Food Sci. Emerg. Technol. 2010; 11, 592–597.
33. Jalté M., Lanoisellé J.-L., Lebovka N. I., Vorobiev E. Freezing of potato tissue pre-treated by pulsed electric fields. LWT – Food Sci. Technol. 2009; 42, 576–580.
34. Saulis G. Electroporation of cell membranes: The fundamental effects of pulsed electric fields in food processing. Food Eng. Rev. 2010; 2, 52–73.
35. Goldberg E., Suárez C., Alfonso M., Marchese J. A. Soba and G. Marshall, Cell membrane electroporation modeling: A multiphysics approach. Bioelectrochemistry 2018; 124, 28–39.
36. Napotnik T. B., Reberšek M., Vernier P. T., Mali B., Miklavčič D. Effects of high voltage nanosecond electric pulses on eukaryotic cells (in vitro): A systematic review. Bioelectrochemistry 2016; 110, 1–12.
37. Davison B. H., Parks J., Davis M. F., Donohoe B. S. Plant Cell Walls. UK: John Wiley & Sons, Ltd. 2013.
38. Armstrong C. M., Hille B. Voltage-gated ion channels and electrical excitability. Neuron 1998; 20, 371–380.
39. Vorobiev E., Lebovka N. Enhanced extraction from solid foods and biosuspensions by pulsed electrical energy. Food Eng. Rev. 2010; 2, 95–108.
40. Buckow R., Baumann P., Schroeder S., Knoerzer K. Effect of dimensions and geometry of co-field and co-linear pulsed electric field treatment chambers on electric field strength and energy utilisation. J. Food Eng. 2011; 105, 545–556.
41. Pataro G., Ferrari G., Donsi F. Mass transfer enhancement by means of electroporation. In: Marko J. (Edit.) Mass Transfer in Chemical Engineering Processes 2011; 151–176, InTech.
42. Góngora-Nieto M. M., Pedrow P. D., Swanson B. G., Barbosa-Cánovas G. V. Energy analysis of liquid whole egg pasteurized by pulsed electric fields. J. Food Eng. 2003; 57, 209–216.
43. Puértolas E., López N., Condón S., Álvarez I., Raso J. Potential applications of PEF to improve red wine quality. Trends Food Sci. Technol. 2010; 21, 247–255.
44. Tintchev F., Dobreva A., Schulz H., Toepfl S. Effect of pulsed electric fields on yield and chemical composition of rose oil (Rosa damascena Mill.). J. Essent. Oil-Bear Plants 2012; 15, 876–884.
45. Eing C., Bonnet S., Pacher M., Puchta H., Frey W. Effects of nanosecond pulsed electric field exposure on Arabidopsis thaliana. IEEE Trans Dielectr. Electr. Insul. 2009; 16, 1322–1328.
46. Boussetta N., Soichi E., Lanoisellé J.-L., Vorobiev E. Valorization of oilseed residues: Extraction of polyphenols from flaxseed hulls by pulsed electric fields. Ind. Crops Prod. 2014, 52, 347–353.
47. Toepfl S. Pulsed electric field food treatment – scale up from lab to industrial scale. Procedia Food Sci. 2011; 1, 776–779.
48. Lebovka N. I., Mhemdi H., Grimi N., Bals O., Vorobiev E. Treatment of potato tissue by pulsed electric fields with time-variable strength: Theoretical and experimental analysis. J. Food Eng. 2014; 137, 23–31.
49. Hejazian M., Phan D.-T., Nguyen N.-T. Mass transport improvement in microscale using diluted ferrofluid and a non-uniform magnetic field. RSC Adv. 2016; 6, 62439– 62444.
50. Gülçin İ., Elmastaş M., Aboul-Enein H. Y. Antioxidant activity of clove oil – A powerful antioxidant source. Arabian J. Chem. 2012; 5, 489–499.
51. Damayanti R., Batubara I., Suparto I. H. Essential oil of red galangal (Alpinia galanga (L) Willd) rhizomes as slimming aromatherapy. Int. J. Pharma Bio Sci. 2015; 6, 283–289.
52. Sujono H., Budiman S., Fudiesta Y., Sahroni A., Jasmansyah J., Khumaisah L. L. Antifungal activity of red galangal oil (Alpinia purpurata K. Schum) against Malassezia furfur. J. Kartika Kimia 2019; 2, 86–91.
53. Darwis S. N., Indo A. M. and Hasiyah S. Tumbuhan obat famili Zingiberaceae [Zingiberaceae Family Medicinal Plants]. Bogor: Center for Industrial Plant Research and Development 1991.
54. Juergens U. Anti-inflammatory properties of the monoterpene 1.8-cineole: Current evidence for co-medication in inflammatory airway diseases. Drug Res. 2014; 64, 638–646.
55. Yajun Z., Changmei X., Susu Z., Guangming Y., Ling Z., Shujie W. Effects of high intensity pulsed electric fields on yield and chemical composition of rose essential oil. Int. J. Agric Biol. 2017; 10, 295–301.
56. Dobreva A., Tintchev F., Heinz V., Schulz H., Toepfl S. Effect of pulsed electric fields (PEF) on oil yield and quality during distillation of white oil-bearing rose (Rosa alba L.). Zeitschrift für Arznei – & Gewürzpflanzen 2010; 15, 127–132 (2010).
57. Lin S., Guo Y., Liu J., You Q., Yin Y., Cheng S. Optimized enzymatic hydrolysis and pulsed electric field treatment for production of antioxidant peptides from egg white protein. Afr. J. Biotechnol. 2011; 10, 11648–11657.
58. Bakhshabadi H., Mirzaei H., Ghodsvali A., Jafari S. M., Ziaiifar A. M., Farzaneh V. The effect of microwave pretreatment on some physico-chemical properties and bioactivity of black cumin seeds’ oil. Ind. Crops Prod. 2017; 97, 1–9.
59. Palafox J. O., Navarrete A., Sacramento-Rivero J. C., Rubio-Atoche C., Escoffie P. A., Rocha-Uribe J. A. Extraction and characterization of oil from Moringa oleifera using supercritical CO2 and traditional solvents. Am. J. Anal. Chem. 2012; 3, 946–949.
60. Mahlinda; Supardan M. D. Distillation of patchouli oil using firewood and liquefied petroleum gas as fuel: Effects of yield, quality and cost analyses. J. Phys. Conf. Ser. 2020; 1500, 012059.
61. Paoplook K., Eshtiaghi M. N. Impact of high electric field pulses on cell disintegration and oil extraction from palm fruit mesocarp. Int. J. Agric. Innov. Res. 2013; 2, 363–369.
62. Alam S. N., Pujiarti R., Kasmudjo K. Effect of distillation tank density and storage time on the quality and chemical composition of cajuput oil. Wood Res. J. 2019; 10, 18–25.
63. Florido P. M., Andrade I. M. G., Capellini M. C., Carvalho F. H., Aracava K. K., Koshima C. C., Rodrigues C. E. C., Gonçalves C. B. Viscosities and densities of systems involved in the deterpenation of essential oils by liquid- liquid extraction: New UNIFAC-VISCO parameters. J. Chem. Thermodyn. 2014; 72, 152–160.
64. Ospina J. D., Tovar C. D. G., Flores J. C. M., Orozco M. S. S. Relationship between refractive ındex and thymol concentration in essential oils of Lippia origanoides Kunth. Chil. J. Agric. Anim. Sci. 2016; 32, 127–133.
65. Gachovska T., Cassada D., Subbiah J., Hanna M., Thippareddi H., Snow D. Enhanced anthocyanin extraction from red cabbage using pulsed electric field processing. J. Food Sci. 2010; 75, E323–E329.
66. Sukardi S., Soeparman S., Argo B. D., Irawan Y. S. The effect of pulsed electric field (PEF) on glandular trichome and compounds of patchouli oil (Pogostemon cablin, Benth). J. Nat. Sci. Res. 2013; 3(15), 48–57.
67. Garde-Cerdán T., González-Arenzana L., López N., López R., Santamaría P., López-Alfaro I. Effect of different pulsed electric field treatments on the volatile composition of Graciano, Tempranillo and Grenache grape varieties. Innov. Food Sci. Emerg. Technol. 2013; 20, 91–99.
68. Tzima K., Brunton N. P., Lyng J. G., Frontuto D., Rai D. K. The effect of pulsed electric field as a pre-treatment step in ultrasound assisted extraction of phenolic compounds from fresh rosemary and thyme by-products. Innov. Food Sci. Emerg. Technol. 2021; 69, 102644.
69. Pataroa G., Carulloa D., Ferraria G. Effect of PEF pre-treatment and extraction temperature on the recovery of carotenoids from tomato wastes. Chem. Eng. Trans. 2019; 75, 139–144.
70. Zhang Z.-H., Zeng X.-A., Brennan C., Brennan M., Han Z., Xiong X.-Y. Effects of pulsed electric fields (PEF) on vitamin c and its antioxidant properties. Int. J. Mol. Sci. 2015; 16, 24159–24173.
71. Dobreva A., Tintchev F., Dzhurmansky A., Toepfl S. Effect of pulsed electric fields on distillation of essential oil crops. CHIMIE Biotech. 2013; 66(9), 1255–1260.
72. Tintchev F., Dobreva A., Schulz H., Toepfl S. Effect of pulsed electric fields on yield and chemical composition of rose oil (Rosa damascena Mill.). J. Essent. Oil-Bear Plants 2012; 15 (6), 876–884.
73. Dobreva A., Tintchev F., Heinz V., Schulz H., Toepfl S. Effect of pulsed electric fields (PEF) on oil yield and quality during distillation of white oil-bearing rose (Rosa alba L.). Zeitschrift für Arznei – & Gewürzpflanzen. J. Herbs Spices Med. Plants 2010; 15(3): 127–132.
74. Zulak K. G., Bohlmann J. Terpenoid biosynthesis and specialized vascular cells of conifer defense. J. Integr. Plant Biol. 2010; 52, 86–97.
75. Santos G. K. N., Dutra K. A., Barros R. A., da Câmara C. A. G., Lira D. D., Gusmão N. B., Navarro D. M. A. F. Essential oils from Alpinia purpurata (Zingiberaceae): Chemical composition, oviposition deterrence, larvicidal and antibacterial activity. Ind. Crops Prod. 2012; 40, 254–260.
76. Sirat H. M., Liamen M. R. Chemical constituents of Alpinia purpurata. Pertanika J. Sci. Technol. 1995; 3, 67–71.
77. Das G., Patra J. K., Gonçalves S., Romano A., Gutiérrez- Grijalva E. P., Heredia J. B., Talukdar A. D., Shome S., Shin H.-S. Galangal, the multipotent super spices: A comprehensive review. Trends Food Sci. Technol. 2020; 101, 50–62.
Štítky
Farmacie FarmakologieČlánek vyšel v časopise
Česká a slovenská farmacie
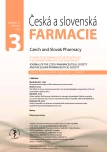
2022 Číslo 3
- Antibiotika na nachlazení nezabírají! Jak můžeme zpomalit šíření rezistence?
- FDA varuje před selfmonitoringem cukru pomocí chytrých hodinek. Jak je to v Česku?
- Jak a kdy u celiakie začíná reakce na lepek? Možnou odpověď poodkryla čerstvá kanadská studie
Nejčtenější v tomto čísle
- Metronomic therapy in the treatment of cancer
- The effect of Aronia melanocarpa extract on the phospholipid composition of the rat myocardium during stress
- Study of possible sex features of ramipril and candesartan treatment under experimental arterial hypertension in rats
- Pulsed electric field energy calculation to damage red galangal (Alpinia purpurata, K. Scumm) rhizome slices and its essential oil yield and quality with hydrodistillation