Design and development of diltiazem hydrochloride transmucosal drug delivery system
Návrh a vývoj systému pro transmukózní přívod diltiazem hydrochloridu
Diltiazem hydrochlorid je antihypertenzní látka, která vykazuje značný first pass metabolismus, a to ji činí vhodným kandidátem pro bukální podání. Mukoadhezivní bukální náplasti byly připraveny s HPMC, chitosanem, PVP, PVA a carbopolem. Fyzikálně-chemické interakce mezi léčivem a polymery byly sledovány pomocí FTIR a DSC: stanovení neprokázala žádné interakce mezi diltiazem hydrochloridem a polymery. U bukálních náplastí se stanovily různé fyzikálně-chemické parametry, uvolňování léčiva in vitro a permeace ex vivo přes bukální sliznici prasete. Zbytkové obsahy rozpouštědel v náplastech se určily plynovou chromatografií a byly pod tolerovanými limity. Při ex vivo studii se léčivo z náplastí uvolňovalo rovnoměrně po dobu 12 hodin kinetickým modelem podle Korsmeyer-Peppase. Stabilita optimalizované formulace se zkoumala podle pokynů ICH a z hlediska obsahu léčiva a jeho permeace ex vivo vykazovala výborné výsledky.
Klíčová slova:
diltiazem hydrochlorid • bukální náplasti • bytková rozpouštědla • mukoadheze, uvolňování léčiva in vitro • permeace ex vivo
Authors:
Subhash Chandra Bose Penjuri; Saritha Damineni; Nagaraju Ravouru
Published in the journal:
Čes. slov. Farm., 2013; 62, 19-27
Category:
Původní práce
Summary
Diltiazem hydrochloride is an antihypertensive agent which undergoes extensive first pass metabolism making it a possible candidate for buccal delivery. Diltiazem mucoadhesive buccal patches were prepared using HPMC, chitosan, PVP, PVA and carbopol. The physicochemical interactions between diltiazem and the polymers were investigated by FTIR and DSC, results revealed no interaction between drug and polymers. The patches were evaluated for various physicochemical parameters, in vitro release studies and ex vivo permeation through porcine buccal mucosa. Residual solvent content in patches was determined by gas chromatography and are largely below the tolerated limits. The formulations showed an extended release of the drug upto a period of 12 hours during ex vivo permeation and showed non Fickian drug release. Stability of the optimized formulation was investigated as per ICH guidelines and was found to be stable with respect to drug content and ex vivo permeation.
Keywords:
diltiazem hydrochloride • buccal patches • residual solvents • mucoadhesion • in vitro drug release • ex vivo permeation
Introduction
Amongst the various routes of drug delivery, the oral route is most preferred to the patient. However, oral administration of drugs has disadvantages such as hepatic first pass metabolism and enzymatic degradation within the GI tract, that prohibit oral administration of certain classes of drugs, especially peptides and proteins. Consequently, other absorptive mucosae are considered as potential sites for drug administration. Transmucosal routes of drug delivery (mucosal linings of nasal, rectal, vaginal, ocular and oral cavity) offers distinct advantages over oral administration for systemic drug delivery. These advantages include possible bypass of first pass effect, avoidances of pre-systemic elimination within the GI tract and better enzymatic flora for drug absorption1–3). Though the rectal, vaginal and ocular mucosa offer certain advantages, the poor patient acceptability associated with these sites renders them reserved for local applications rather than systemic drug administration. The oral cavity, on the other hand, is highly acceptable by the patient, the mucosa is relatively permeable with rich blood supply, it is robust and shows a short recovery time after stress or damage4–6) and the virtual lack of Langerhans cells make the oral mucosa tolerant to potential allergens. Furthermore, oral transmucosal drug delivery bypasses first pass effect and avoids presystemic elimination in the GI tract. These factors make the oral mucosal cavity a very attractive and feasible site for systemic drug delivery.
Diltiazem is an effective calcium channel blocker, used in the treatment of angina, hypertension and myocardial infarction. It was reported to be rapidly absorbed after oral administration, but it undergoes extensive first pass metabolism leading to poor bioavailability (40%). In addition, diltiazem hydrochloride has a low dose (30 mg), low molecular weight (451) and an extensive first pass effect; a need for long term treatment and repetitive dosing7). All these parameters make this drug an interesting candidate for buccal administration.
Experimetal part
Material and methods
Diltiazem hydrochloride (BP) was a gift from Nicholas Piramil (Kohir, India). Chitosan (food grade, deacetylation value: more than 85% and molecular weight: 15000) was a generous gift from Marine Chemicals (Cochin, India); Hypromellose (HPMC K15M: Molecular weight: 10.000–1.500.000; Viscosity: 15.000 cP) and Carbomer (carbopol 934P; Viscosity: 29,400–39,400 cP) was obtained from Tini Pharma Ltd., (Tirupathi, India); Polyvinyl alcohol (Molecular weight: 20000, Viscosity: 4–7 cP) was obtained from Loba Chemie Pvt. (Mumbai, India) and Povidone (Polyvinyl pyrrolidone K30; Molecular weight: 50000, Viscosity: 5.5–8.5 cP) was gifted by the International Specialty Products (Hyderabad, India). All reagents and solvents used were of analytical grade.
Investigation of drug-excipient interactions
Fourier transform infrared spectroscopy
Compatibility between the drug and the polymers was studied by FTIR spectra. FTIR studies were carried out for drug and its physical mixture (1 : 1). The sample was dispersed in KBr powder and the compacts were made by applying 6000 kg/cm2 pressure and analyzed. FTIR spectra were obtained by diffuse reflectance on a FTIR spectrophotometer type FTIR 8400 (Schimadzu Corporation, Japan). The positions of FTIR bands of important functional groups of drug were identified and were cross-checked in obtained spectra.
Differential scanning calorimetry (DSC)
DSC studies for the drug and its physical mixture ( 1: 1) were carried out using a DSC-60 calorimeter (Schimadzu Corporation, Japan). The instrument was calibrated with an indium and zinc standard. The sample was heated from 10 to 300 °C at a heating rate of 25 °C/min to remove thermal history. The sample was then immediately cooled to 10 °C and reheated from 10 to 300 °C under the flow of nitrogen at a heating rate of 10 °C/min.
Preparation of patches
Diltiazem hydrochloride mucoadhesive buccal patches were prepared by solvent casting technique using chitosan, hydroxypropyl methyl cellulose (HPMC K15M), polyvinyl pyrrolidone (PVP K30), polyvinyl alcohol (PVA) and carbopol 934P as polymers. Propylene glycol and dimethyl sulfoxide (DMSO) were used as the plasticizer and penetration enhancer, respectively. Acetic acid, ethanol, methanol and dichloromethane (DCM) were used as solvents. The composition of samples used for the preparation of buccal patches is shown in Table 1. The drug was dissolved in a small quantity of solvent and polymers were dissolved in the remaining solvent/solvent mixture. The drug, polymer solutions along with the plasticizer and permeation enhancer were sonicated for 30 min and examined for air entrapment. The solution was poured onto glass moulds of 10 x 5 cm2 and air-dried for overnight at room temperature. An inverted funnel was kept on the mould for controlled evaporation. The dried film of the drug was peeled from the mould and packed in an aluminium foil and kept in a desiccator till further use.
The backing layer was also prepared by the solvent casting method by dissolving 500 mg ethylcellulose in 15 ml of an ethanol-toluene mixture (1 : 4). The medicated patches were laminated on one side with the backing layer and used for release studies.
Drug content
Drug content of patches was determined by dissolving five patches (1 cm2) in 100 ml of phosphate buffer of pH 6.6. After suitable dilutions the resultant solution was filtered and analysed for diltiazem content spectrophotometrically using a UV-1700 Pharma Spec spectrophotometer, Schimadzu Corporation, Japan at 237 nm.
Thickness and weight variation8, 9)
The thickness of patches was assessed using a micrometer screw gauge (Mitutoyo, Japan). From each formulation, three randomly selected patches with a surface area of 1 cm2 were used. Each patch was weighed individually on an analytical balance (Shimadzu, Japan) and the average weights were calculated.
Folding endurance10)
Folding endurance of patches was determined manually by repeatedly folding a film at the same place until it breaks. The number of foldings required to break or crack a patch was taken as the folding endurance.
Surface pH11)
Patches were placed in Petri dishes containing 5 ml phosphate buffer (pH 6.6) and the pH at the surface was measured by placing the tip of the glass microelectrode of a digital pH meter (Elico LI 120, India) close to the surface of the patch and allowing it to equilibrate for 1 min prior to recording. Experiments were performed in triplicate.
Swelling index12)
Swelling index of the patches was evaluated by placing them in Petri dishes containing 4 ml of phosphate buffer pH 6.6 at room temperature. The patches were taken at regular intervals from the Petri dish and excess buffer was removed using filter paper. The swollen system was reweighed (w2). The difference between the initial weight (w1) and the weight gained at regular time interval (w2) was used to determine the swelling index which was calculated as S.I. = (w2 – w1/w1) x 100.
Preparation of porcine buccal mucosa13)
Buccal tissue was obtained from a local slaughterhouse from a freshly sacrificed porcine and used within 3 to 4 hr of sacrifice. The tissue was stored in isotonic phosphate buffer (pH 7.4) at 4 ± 1 °C upon collection. The epithelium was separated from the underlying connective tissue using a surgical blade and the membrane was used for the experiments.
In vitro residence time14)
In vitro residence time was determined according to the method described by Nafee et al. The apparatus consists of a disintegration apparatus with 800 ml of phosphate buffer pH 6.6 maintained at 37 ± 1 °C . Porcine buccal mucosa was glued to the glass slide and held vertically in the apparatus. The buccoadhesive patch was hydrated with 0.5 ml of phosphate buffer pH 6.6 and the hydrated surface was brought in contact with the buccal mucosa and pressed by applying pressure with the thumb for 5 min. The glass slide was allowed to move up and down (28–32 strokes per minute) so that the patch was completely immersed in the buffer solution at the lowest point and was out at the highest point. The time required for the complete erosion or detachment of the patch from the mucosal surface was recorded.
In vitro mucoadhesion test
In vitro bioadhesion of the patches was determined by the procedure of Varsha et al.15) using porcine buccal mucosa. A piece of porcine buccal mucosa was cut and glued with a commercially available adhesive on the ground surface of a tissue holder made of thin plastic sheet. Similarly, the patch was glued to another tissue holder of the same size. Then the tissue holders with porcine buccal mucosa and patch were put in contact with each other by applying constant pressure with the thumb for 5 min to facilitate adhesion. The tissue holder with porcine buccal mucosa was allowed to hang on an iron stand with the help of an aluminium wire fastened with the hook provided on the back of the holder. A preweighed lightweight polypropylene bottle was attached to the hook on the back side of the formulation holder with aluminium wire. After a preload time of 5 min, water was added to the polypropylene bottle through an intravenous infusion set at a constant rate. The addition of water was stopped when the buccoadhesive system was detached from buccal mucosa. The weight required to detach the system from buccal mucosa was noted.
The force of adhesion and the bond strength14) were calculated as:
Weight (g) x 9.81
Force of adhesion (N) = ––––––––––––––
1000
Force of adhesion (N)
Bond strength (N/m2) = –––––––––––––––––––––
Surface area of patch (m2)
Determination of residual solvents
Methanol16), ethanol17), dichloromethane18) and acetic acid19) content in patches was determined by gas chromatography on an Agilent 7890 Gas Chromatograph, USA, fitted with a flame ionization detector. For estimation of residual solvents, 1 cm2 patch was dissolved in a small amount of DMSO in a 10 ml volumetric flask and the volume was made up to 10 ml with DMSO. The solution was filtered through a 0.45 μm filter and degassed using a sonicator. From the sample, 1 μl was injected into the injection port, the chromatogram was recorded and the peak area of the solvent was measured. The concentration of the residual solvent was calculated from calibration curve data.
In vitro release studies15)
The apparatus consists of a receptor compartment (250 ml beaker), which is covered with a thin plastic sheet with three holes, one for a thermometer, second for a sample collection tube and third for a formulation holding pelete glass rod shown in Fig. 1. Before starting the in vitro study, the patch was attached to the glass rod and placed four inches above the receptor. The dissolution medium was 100 ml of phosphate buffer pH 6.6. The temperature was maintained at 37 ± 1 °C on a heat-controlled hot plate with a magnetic stirrer. Dissolution fluid was stirred at a constant speed of 50 rpm using a magnetic bead. Samples were withdrawn at regular intervals and the same volume of fresh phosphate buffer pH 6.6 was replaced into the beaker to maintain the constant amount of dissolution medium. The samples were filtered through a 0.45 μm filter paper (Millipore) and drug concentration was analyzed spectrophotometrically. The experiment was carried out in triplicate for each formulation and the mean release was determined.
HPLC analysis20)
Analysis of diltiazem was performed using a Schimadzu LC -2010CHT HPLC system equipped with a UV detector and an Inertsil column (150 x 4.6 mm i.d) at ambient temperature. The mobile phase was a mixture of water and acetonitrile (63 : 37) containing 0.35% w/v of triethylamine and the pH was adjusted to 3.0 with 5% orthophosphoric acid. The solution was filtered through a 0.45 μm filter and degassed by sonication. The flow rate was 1 ml per minute. Detection was carried on at 240 nm wavelength. A calibration curve was plotted for diltiazem in the range of 10–50 μg/ml. Good linear relationship was observed between the concentration of diltiazem and its peak area (r2 = 0.997). Precision and accuracy of the HPLC method were estimated.
Ex vivo permeation studies21)
One cm2 patch under study was placed in intimate contact for five minutes with the excised porcine buccal mucosa and mounted between the two compartments of Franz diffusion cell. A teflon bead was placed in the receptor compartment filled with 25 ml of pH 6.6 phosphate buffer. The diffusion cell was thermostated at 37 ± 1 °C and at a rate of 50 rpm. The samples were withdrawn at regular intervals and the same volume of fresh phosphate buffer pH 6.6 was replaced into the diffusion cell to maintain a constant amount of diffusion medium. Samples were filtered through a 0.45 μm filter paper (Millipore) and analyzed for drug content using HPLC and the data were statistically analysed by one way ANOVA followed by turkey post hoc test for multiple comparison using graph pad prism. Differences were considered to be significant at a level of p < 0.05.
The permeability coefficients (P) were calculated as follows22):
P = (dQ/dt)/ (CA),
where: dQ/dt – permeation rate, C – concentration of the donor chamber, A – surface area of diffusion.
Steady state fluxes (Jss) were calculated by dividing the slope of the cumulative amount permeated vs. time curve by the diffusional area.
Drug release from backing layer23)
A 1 cm2 patch was placed between the two compartments of a Franz diffusion cell at 37 ± 1 °C with the backing layer facing the receptor compartment filled with 25 ml of pH 6.6 phosphate buffer. The samples were withdrawn at regular intervals and the same volume of fresh phosphate buffer pH 6.6 was replaced into the diffusion cell to maintain the constant amount of the diffusion medium. Samples were filtered and analyzed for drug content.
Stability in artificial saliva24, 25)
Stability of the patches was assessed in artificial saliva. Patches were placed in Petri dishes containing 5 ml of artificial saliva (composition: Sodium carboxy-methylcellulose 10 g, potassium chloride 0.625 g, magnesium chloride 0.059 g, calcium chloride 0.166 g, di potassium hydrogen phosphate 0.804 g, potassium dihydrogen phosphate 0.326 g, methyl p-hydroxybenzoate 2 g and water up to 1000 ml) and kept in a temperature controlled oven at 37 ± 1 °C for 6 h. The patches were examined for changes in texture and drug content.
Stability studies26)
Stability studies were conducted according to the ICH Q1A (R2) guidelines. Patches were wrapped in an aluminum foil and were kept in a stability chamber at a temperature of 40 ± 2 °C and 75 ± 5% RH for 6 months. Samples were withdrawn at the end of 6 months and analyzed for drug content and ex vivo permeation through porcine buccal mucosa. The zero time samples were used as control for the study and the results were statistically analyzed by using t-test and p < 0.05 were considered as significant.
Results and discussion
Investigation of drug-excipient interactions
FTIR spectral analysis
Diltiazem hydrochloride pure drug and with excipients (F1–F4, Table 1) were subjected to FTIR spectroscopic analysis. The obtained spectra are shown in Fig. 2. Characteristic peaks of diltiazem were compared with the peaks obtained with the physical mixture. The FTIR spectra of pure diltiazem showed sharp characteristic peaks at 781, 839 (C–H out-of-plane), 1679, 1743 (C = O stretch), 2395 (N–H stretch) and 2922, 2971 cm-1 (C–H stretch). All the above characteristic peaks appeared in the spectra of physical mixtures at the same wavenumbers indicating no modification or interaction between the drug and polymers.
Differential scanning calorimetry
DSC studies were carried out for diltiazem hydrochloride pure drug and with excipients (F1–F4, Table 1). DSC thermograms obtained are shown in Fig. 3. The DSC thermogram of diltiazem showed an endothermic peak at 214.74 °C corresponding to its melting temperature, which was also detected in the thermograms of the physical mixture, signifying no interaction between diltiazem and the polymers.
Physicochemical evaluation of diltiazem buccal patches
Physicochemical evaluation data are shown in Table 2. The drug content was determined in triplicate and it was found to be uniform in all the patches. The patches (F1 to F4) were weighing in between 27.09 mg to 29.85 mg. Patch thickness was in the range of 257 μm to 323 μm. Folding endurance of the patches was in the following order F1 > F4 > F3 > F2. The folding endurance of all the films was optimal, the films exhibited good physical and mechanical properties. High alkaline or acidic pH of patches may cause irritation to the buccal mucosa and influence the degree of hydration of polymers27, 28), so the surface pH of patches was determined to optimize release and adhesion. The surface pH of all formulations was in the range of 5.9–7 pH, i.e. close to buccal pH. The swelling index of the patches was in the order F1 > F4 > F2 > F3 and showed significant difference. Formulation F1 showed the highest swelling which may be due to the presence of highly swellable HPMC. Formulations F2 and F3 showed faster swelling (with in 45 min) compared to other formulations due to more hydrophilic nature of PVP and PVA29). The high initial uptake of water was observed in F1, F2 and F3 due to a faster hydration rate of polymers. Carbopol will undergo ionization at pH 6.8 and leads to the generation of negative charges at the backbone of the polymer. Repulsion between these like charges leads to uncoiling of the polymer to produce an extended structure capable of greater uptake of water23, 30), which leads to the greater swelling index of F4.
In vitro residence time of the patches was in the order F4 > F1 > F2 > F3 and showed significant difference. A higher residence time in F4 may be due to the presence of carbopol with higher mucoadhesivity than those of other polymers31). Whereas the presence of PVP and PVA (hydrophilic polymers) leads to a decrease in the residence time of the patches29, 32). Between F2 and F3, formulation F2 showed a longer residence time which may be due to a greater hydrophilic nature of PVP than that of PVA. And also mucin contains 95% of water32), so PVP showed more affinity towards mucin. The results are in agreement with earlier reports of Hao et al.33), Nafee et al.34) and Patel et al.35).
An effective buccal mucosal delivery device must maintain intimate contact with the mucus layer overlying the epithelial tissue. This parameter is critical for successful utilization of these dosage forms. Hence, in vitro mucoadhesion testing was performed using the pork mucosal membrane, which gives an indirect measurement of the bioadhesive strength in grams. Table 3 shows the in vitro bioadhesive strength of diltiazem patches, the bond strength values were found to be 951.9, 868.87, 831.56 and 1051.76 N/m2 for F1, F2, F3 and F4, respectively, and significant difference was found in bioadhesive strength of patches. F4 showed the greatest bioadhesive strength followed by F1, F2 and F3. Formulations F4 and F1 showed greater bioadhesive strength due to the presence of carbopol and HPMC, respectively36).
According to ICH guidelines, acetic acid and ethanol are class III solvents (solvents with low toxic potential) and the limit of 5000 ppm is acceptable without justification. Methanol, dichloromethane and toluene are class II solvents (solvents to be limited) thus the limits of 3000 ppm, 600 ppm and 890 ppm, respectively, are acceptable. The residual solvents contents in patches are largely below the tolerated limits (Table 4).
In vitro release studies
In vitro release studies of patches were carried out in triplicate. After 6 h the release was found to be 81.84 ± 2.26, 87.46 ± 2.9, 86.9 ± 1.83 and 79.7 ± 2.36% for the formulations F1, F2, F3 and F4, respectively (Fig. 4). The data of in vitro release were analyzed by one way ANOVA and there was no significant difference between means at 30 & 90 minutes. In vitro release studies clearly showed that the percent release of diltiazem was maximum, i.e. 87.46% for F2. The order of drug release was found to be F2 > F3 > F1 > F4. All the drug dissolution profiles showed zero order kinetic models with non-Fickian diffusion mechanism.
Ex vivo permeation studies
Ex vivo permeation studies for the patches were carried out in triplicate and after 12 hours the release was found to be 76.38 ± 5.11, 84.21 ± 6.42, 80.37 ± 5.03 and 73.71 ± 4.27% for the formulations F1, F2, F3 and F4, respectively (Fig. 5). The data of ex vivo permeation were analyzed by one way ANOVA and significant difference was not observed between means up to 360 minutes. In the ex vivo permeation study, formulation F2 showed the maximum release of the drug, 84.21% in 720 min; this formulation was considered as the optimized one and used for further study. The order of drug release is F2 > F3 > F1 > F4. F2 showed a greater release followed by F3 which may be due to the presence of hydrophilic polymers PVP and PVA, respectively, which absorb more water and promote dissolution. Moreover, PVP will dissolve and create channels for the drug to diffuse from the patches29). Though F1 showed a greater swelling index, it showed a slow release of diltiazem from the patches, which may be due to high viscous nature of HPMCK15M; a thick gel (diffusion path length) was formed and it acts as a barrier for drug diffusion and slow erosion of the polymer37). F4 also showed a slow release, which may be due to the formation of a thick gel barrier by chitosan and carbopol29, 31).
The drug release data obtained were fitted into the zero order, first order, Higuchi and Korsmeyer-Peppas equations to know the mechanism of drug release from these formulations. The ex vivo permeation profile of F1 and F3 formulations could be best expressed by the Korsmeyer-Peppas model, as the plots showed the highest linearity (r2: 0.9948 and 0.994, respectively), and F2 and F4 formulation could be best expressed by the zero order model, as the plots showed the highest linearity (r2: 0.9968 & 0.9963, respectively). All the formulations showed a non-Fickian release pattern (Table 5) as it was evidenced from the release exponent (n > 0.5), which indicates coupling of the diffusion and erosion mechanism, called anomalous diffusion, and shows that the drug release is controlled by more than one process. So, the suggested drug release mechanism for diltiazem patches may be a combination of diffusion and erosion of the polymer matrix.
The time taken for the permeation of 50% diltiazem was found to be 430, 401, 408 and 466 minutes for the formulations F1, F2, F3 and F4, respectively. The mean steady state flux (Jss) was found to be 0.666 ± 0.05, 0.7149 ± 0.027, 0.6929 ± 0.016, 0.6269 ± 0.04 mg/cm2/hr and the permeability coefficient was found to be 0.0666 ± 0.005, 0.0715 ± 0.003, 0.0693 ± 0.002, 0.0623 ± 0.004 cm/hr (Table 6) for the formulations F1, F2, F3 and F4, respectively.
Drug release from backing layer
Drug release from the backing layer was investigated to ascertain the efficiency of the backing membrane to provide unidirectional release of the drug through the patch. The results of the study showed that no drug was released during the study.
Stability studies
The stability study of F2 was conducted in artificial saliva to mimic the stability of the drug and the formulation in the oral cavity. No color change was observed. Thickness of patches increased to 18.15% owing to swelling in artificial saliva over 6 h (Table 7). The recovery of drug from the patches was 99.5% (9.92 mg) indicating maximum utilization of the drug incorporated.
Accelerated stability studies were performed for optimized formulation (F2) as per ICH Q1A (R2) guidelines at 40 ± 2 °C and 75 ± 5% RH for 6 months. After specified duration, visual examination of the buccal patches did not show any change in morphology. The values of drug content, t50%, flux and permeability coefficient of optimized formulation before and after stability study are depicted in Table 8.
The results of the stability studies revealed that there was a significant change in drug content and ex vivo permeation through porcine buccal mucosa. Shelf life of the formulation was calculated by using “Stab R” 38) software and it was found to be 22 months. The cumulative percentage of diltiazem permeated in 12 h was found to be 79.86 ± 3.15%. Flux and permeability coefficient of diltiazem was found to be 0.6913 ± 0.024 mg/cm2/hr and 0.0691 ± 0.002 cm/hr, respectively, for the optimized formulation after stability study.
The ex vivo permeation profile of F2 after stability study could be best expressed by zero order model, as the plots showed highest linearity (r2: 0.9932) and the obtained release exponent (n) value, 0.9375, supported non Fickian release. From the results, it was observed that there was no change in the best fit model and transport mechanism even after stability study.
Conclusion
A novel mucoadhesive buccal patch of diltiazem hydrochloride with unidirectional drug delivery was developed to overcome the first-pass metabolism and subsequent low bioavailability of the drug. The bioadhesive patch displayed sufficient bioadhesive strength and in vitro drug release. The ex vivo permeation studies have shown that this is a potential drug delivery system for diltiazem hydrochloride with considerably good stability and release profile. The release of drug was found to be a combination of diffusion and erosion of polymers. So, it is possible to formulate mucoadhesive patches of diltiazem hydrochloride with the intention of obtaining better therapeutic efficiency by sustaining drug release thereby improving patient compliance and increasing bioavailability with decreased dosing.
Conflicts of interest: none.
Received 27 November 2012 / Accepted 7 Januar 2013
Subhash Chandra Bose Penjuri (✉ )
Department of Pharmaceutics, MNR College of Pharmacy
Sangareddy-502294
Andhra Pradesh, India
e-mail: penjurisubhash@gmail.com
Saritha Damineni
Department of Pharmaceutics, Sultan-ul-uloom College of Pharmacy, Hyderabad, India.
Nagaraju Ravouru
Institute of Pharmaceutical Technology, Sri Padmavathi Visva Vidyalayam, Tirupathi, India
Zdroje
1. Aungst B. J., Rogers M. J., Shefter E. Comparison of nasal, rectal, buccal, sublingual and intramuscular Insulin efficacy and the effects of a bile salt as absorption promoter. The J Pharmacol Exp Ther. 1998; 244, 23–27.
2. Lee W. E. Permeation enhancers for nasal drug delivery of protein and peptide therapeutics. Biopharm. 1990; 3, 22–25.
3. Soyani A. P., Chein Y. W. Systemic delivery of peptides and proteins across absorptive mucosae. Crit Rev Therapy: Drug Carries System. 1996; 13, 85–184.
4. Rathbone M. J., Hadgraft J. Absorption of drugs from the human oral cavity. Int J Pharm. 1991; 74, 9–24.
5. Squier C. A. The permeability of oral mucosa. Crit Rev Oral Biol Med. 1991; 2, 13–32.
6. Gandhi R. E., Robinson J. R. Bioadhesion in drug delivery. Ind J Pharm Sci. 1998; 50, 145–152.
7. Saisavam S., Muhammed M. H. Design and evaluation of Diltiazem Hydrochloride buccal patches. Ind J Pharm Sci. 2000; 62, 236–238.
8. Verma P. R. P., Sunil S. I. Transdermal Delivery of Propranolol Using Mixed Grades of Eudragit: Design and in vitro and in vivo Evaluation. Drug Dev Ind Pharm. 2000; 26, 471–476.
9. Pavankumar G. V., Ramakrishna V., William G. J., Konde A. Formulation and evaluation of buccal films of Salbutamol sulphate. Indian J Pharm Sci. 2005; 67, 160–164.
10. Khanna R., Agarwal S. P., Ahuja A. Preparation and evaluation of buccal films of clotrimazole for oral candida infections. Indian J Pharm Sci. 1997; 59, 299–305.
11. Nafee N. A., Boraie N. A., Ismail F. A., Mortada L. M. Mucoadhesive delivery systems II. Formulation and in vitro/ in vivo evaluation buccal mucoadhesive tablets containing water soluble drugs. Drug Dev Ind Pharm. 2004; 30, 995–1004.
12. Ilango R., Kavimani S., Mullaichasam A. R., Jayakar B. In vitro studies on buccal strips of Glibenclamide using chitosan. Indian J Pharm Sci. 1997; 59, 232–235.
13. Kulkarni U., Mahalingam R., Pather S. I., Li X., Jasti B. Porcine Buccal Mucosa as an In Vitro Model: Relative Contribution of Epithelium and Connective Tissue as Permeability Barriers. J Pharm Sci. 2009; 98, 471–483.
14. Metia P. K., Bandyopadhyay A. K. In vitro Evaluation of Novel Mucoadhesive Buccal Tablet of Oxytocin Prepared with Diospyros Peregrina Fruits Mucilages, Yakugaku Zasshi. 2008; 128, 603–609.
15. Agarwal V., Mishra B. Design, Development, and Biopharmaceutical Properties of Buccoadhesive Compacts of Pentazocine. Drug Dev Ind Pharm. 1999; 25, 701–709.
16. Puranik S. B., Varun Pawar R., Lalitha N., Pai P. N., Rao G. K. Residual Solvent Analysis in Hydrochloride Salts of Active Pharmaceutical Ingredients. Pak J Pharm Sci. 2009; 22, 410–414.
17. Stackler B., Christensen E. N. Quantitative Determination of Ethanol in Wine by Gas Chromatography. Amer J Enol Viticult. 1974; 25, 202–207.
18. Robert Schubert B. S., Ketel L. Gas Chromatographic Determination of Methylene Chloride in Aerosol air Sprays. J Soc Cosmet Chem. 1972; 23, 115–124.
19. Kratochvil J., Holas J. A gas chromatographic method for the determination of acetic acid and lactic acid in rye sour. Cereal Chem. 1980; 57, 209–211.
20. Al Saidan S. M., Krishnaiah Y. S., Patro S. S., Satyanaryana V. In Vitro and In Vivo Evaluation of Guar Gum Matrix Tablets for Oral Controlled Release of Water-soluble Diltiazem Hydrochloride. AAPS Pharm Sci Tech. 2005; 6 (1) Article 5.
http://www.aapspharmscitech.org.
21. Patel V. M., Prajapati B. G., Patel J. K., Patel M. M. Design and characterization of chitosan-containing mucoadhesive buccal patches of propranolol hydrochloride. Acta Pharm. 2007; 57, 61–72.
22. Shojaei A. H., Zhou S. L., Li X. Transbuccal Delivery of Acyclovir (II): Feasibility, System Design, and In Vitro Permeation Studies. J Pharm Pharmaceut Sci. 1998; 1, 66–73.
23. Maurya S. K., Bali V., Pathak K. Bilayered transmucosal drug delivery system of pravastatin sodium: Statistical optimization, in vitro, ex vivo, in vivo and stability assessment. Drug Delivery. 2012; 19, 45–57.
24. Vasantha P. V., Puratchikody A., Mathew S. T., Balaraman A. K. Development and characterization of Eudragit based mucoadhesive buccal patches of salbutamol sulfate. Saudi Pharmaceutical Journal. 2011; 19, 207–214.
25. Palem C. R., Gannu R., Doodipala N., Yamsani V. V., Yamsani M. R. Transmucosal Delivery of Domperidone from Bilayered Buccal Patches: In Vitro, Ex Vivo and In Vivo Characterization. Arch Pharm Res. 2011; 34, 1701–1710.
26. Saranjit Singh. Drug stability testing and shelf-life determination according to international guidelines. Pharmaceutical technology. 1999; 23, 68–88.
27. Chang H. S., Park H., Robinson J. R. Bioadhesive polymers as platform for oral controlled drug delivery: II, synthesis and evaluation of some swelling, water insoluble bioadhesive polymers. J. Pharm. Sci. 1985; 74, 399–405.
28. Park H., Robinson J. R. Physico chemical properties of water insoluble polymers important to mucin epithelial adhesion. J. Control. Rel. 1985; 2, 47–57.
29. Shidhaye S. S., Saindane N. S., Sutar S., Kadam V. Mucoadhesive Bilayered Patches for Administration of Sumatriptan Succinate. AAPS PharmSciTech. 2008; 9, 1–8.
30. Sakellariou P. A study of the leaching/retention of watersoluble polymers in blends with ethylcellulose using torsional braid analysis. J. Control. Rel. 1998; 7, 147–157.
31. Nappinnai M., Chandanbala R., Balaijirajan R. Formulation and Evaluation of Nitrendipine Buccal Films. Indian J Pharm Sci. 2008; 70, 631–635.
32. Satishbabu B. K., Srinivasan B. P. Preparation and Evaluation of Buccoadhesive Films of Atenolol. Indian J Pharm Sci. 2008; 70, 175–179.
33. Hao J. S., Chan L. W., Shen Z. X., Heng P. W. Complexation between PVP and Gantrez polymer and its effect on release and bioadhesive properties of the composite PVP/Gantrez films. Pharm Dev Technol. 2004; 9, 379–386.
34. Nafee N. A., Boraie N. A., Ismail F. A., Mortada L. M. Mucoadhesive buccal patches of miconazole nitrate: in vitro/in vivo performance and effect of ageing. Int J Pharm. 2003; 264, 1–14.
35. Patel V. M., Prajapati B. G., Patel M. M. Effect of hydrophilic polymers on buccoadhesive eudragit patches of Propranolol hydrochloride using factorial design. AAPS PharmSciTech. 2007; 8 (2) Article 45. http://www.aapspharmscitech.org.
36. Chary C. B., Vani G., Rao Y. M. In vitro and in vivo adhesion testing of mucoadhesive drug delivery systems. Drug Dev Ind Pharm. 1999; 25, 685–690.
37. Yehia S. A., El Gazayerly O. N., Basalious E. B. Fluconazole Mucoadhesive Buccal Films: In vitro/In Vivo Performance. Curr Drug Deliv. 2009; 6, 17–27.
38. Lee H. Y., Wu P. C., Lee Y. J. Stab: An R package for drug stability data analysis. Compu methods and programs in biomed. 2010; 100, 140–148.
Štítky
Farmacie FarmakologieČlánek vyšel v časopise
Česká a slovenská farmacie
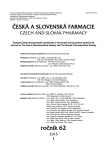
2013 Číslo 1
- Přerušovaný půst může mít významná zdravotní rizika
- Ukažte mi, jak kašlete, a já vám řeknu, co vám je
- Může slepičí polévka opravdu přispívat k uzdravení? Babiččin všelék z pohledu moderní vědy
Nejčtenější v tomto čísle
- Pediatric oral solutions with propranolol hydrochloride for extemporaneous compounding: the formulation and stability study
- Oral mucosa and therapy of recurrent aphthous stomatitis
- Organization and management of nationalized pharmaceutical industry in Slovakia from 1945 to 1948
- Mucoadhesive films as perspective oral dosage form