New insights into the pathophysiology of Ph-negative myeloproliferative neoplasms
Authors:
B. Kráľová; K. Hlušičková Kapraľová; V. Divoký; M. Horváthová
Authors‘ workplace:
Ústav biologie, LF UP v Olomouci
Published in:
Transfuze Hematol. dnes,27, 2021, No. 3, p. 208-217.
Category:
Review/Educational Papers
doi:
https://doi.org/10.48095/cctahd2021208
Overview
Myeloproliferative neoplasms (MPNs) represent a group of related clonal haematological disorders with overlapping phenotypes. The main typical features are excessive production of fully differentiated myeloid cells, chronic inflammation and a tendency to transform to acute myeloid leukaemia. Clonal proliferation in MPN is driven by various somatic mutations, most notably involving Janus kinase 2 (JAK2). However, MPN phenotypic diversity cannot be explained only by cooperation of acquired driver mutations with additional somatic mutations detected in MPN patients. Indeed, MPN initiation and clinical phenotype is a product of complex interactions involving both genetic and non-genetic factors. Recently, genetic predisposition appeared as an important determinant of MPN pathophysiology, particularly of clonal expansion. This review provides insights into complex, newly emerging factors contributing to Philadelphia chromosome (Ph) -negative MPN pathobiology.
Keywords:
myeloproliferative neoplasms – JAK2 – CALR – MPL – genetic predisposition – MPN heterogeneity
Sources
- Dameshek W. Some specultation on the myeloproliferative syndromes. Blood. 1951; 6: 372–375.
- Rumi E, Cazzola M. Diagnosis, risk stratification, and response evaluation in classical myeloproliferative neoplasms. Blood. 2017; 129: 680–692.
- Arber DA, Orazi A, Hasserjian R, et al. The 2016 revision to the World Heath Organization classification of myeloid neoplasms and acute leukemia. Blood. 2016; 127: 2391–2405.
- Kralovics R, Stockton DW, Prchal JT. Clonal hematopoiesis in familial polycythemia vera suggests the involvement of multiple mutational events in the early pathogenesis of the disease. Blood. 2003; 102: 3793–3796.
- Landgren O, Goldin LR, Kristinsson SY, Helgadottir EA, Samuelsson J, Bjorkholm M. Increased risks of polycythemia vera, essential thrombocythemia, and myelofibrosis among 24,577 first-degree relatives of 11,039 patients with myeloproliferative neoplasms in Sweden. Blood. 2008; 112: 2199–2204.
- Rumi E, Passamonti F, Della Porta, MG, et al. Familial chronic myeloproliferative disorders: clinical phenotype and evidence of disease anticipation. J Clin Oncol. 2007; 25: 5630– 5635.
- Tegg EM, Thomson RJ, Stankovich JM, et al. Anticipation in familial hematologic malignancies. Blood. 2011; 117: 1308–1310.
- Nowell PC, Hungerford DA. Chromosome studies on normal and leukemic human leukocytes. J Natl Cancer Inst. 1960; 25: 85–109.
- Ren, R. Mechanisms of BCR-ABL in the pathogenesis of chronic myelogenous leukaemia. Nat Rev Cancer. 2005; 5: 172–183.
- Nieborowska-Skorska M, Kopinski PK, Ray R, et al. Rac2-MRC-cIII-generated ROS cause genomic instability in chronic myeloid leukemia stem cells and primitive progenitors. Blood. 2012; 119: 4253–4263.
- Baxter E, Scott L, Cambell P, et al. Acquired mutation of the tyrosine kinase JAK2 in human myeloproliferative disorder. Lancet. 2005; 365: 1054–1061.
- Kralovics R., Passamonti F., Buser A, et al. A gain-of-function mutation of JAK2 in myeloproliferative disorders. N Engl J Med. 2005; 352: 1779–1790.
- James C, Ugo V, Le Couedic J, et al. A unique clonal JAK2 mutation leading to constitutive signalling causes polycythaemia vera. Nature. 2005; 434: 1144–1148.
- Levine RL, Wadleigh M, Cools J, et al. Activating mutation in the tyrosine kinase JAK2 in polycythemia vera, essential thrombocythemia, and myeloid metaplasia with myelofibrosis. Cancer Cell. 2005; 7 (4): 387–397.
- Klampfl T, Gisslinger H, Harutyunyan AS, et al. Somatic mutations of calreticulin in myeloproliferative neoplasms. N Engl J Med 2013; 369: 2379–2390.
- Nangalia J, Massie CE, Baxter EJ, et al. Somatic CALR mutations in myeloproliferative neoplasms with nonmutated JAK2. N Eng J Med. 2013; 369: 2391–2405.
- Defour JP, Chachoua I, Pecquet C, Constantinescu SN. Oncogenic activation of MPL/thrombopoietin receptor by 17 mutations at W515: implications for myeloproliferative neoplasms. Leukemia. 2016; 30: 1214–1216.
- Pardanani AD, Levine RL, Lasho T, et al. MPL515 mutations in myeloproliferative and other myeloid disorders: a study of 1182 patients. Blood. 2006; 108: 3472–3476.
- O‘Sullivan J, Mead AJ. Heterogeneity in myeloproliferative neoplasms: Causes and consequences. Adv Biol Regul. 2019; 71: 55–68.
- Van Egeren D, Escabi J, Nguyen M, et al. Reconstructing the lineage histories and differentiation trajectories of individual cancer cells in myeloproliferative neoplasms. Cell Stem Cell. 2021; 28: 514–523.
- Williams N, Lee J, Moore L, et al. Phylogenetic reconstruction of myeloproliferative neoplasm reveals very early origins and lifelong evolution. bioRxiv; publikováno elektronicky 9. novembra 2020. doi: 10.1101/2020.11.09.374710.
- Jatiani SS, Baker SJ, Silverman LR, Reddy EP. JAK/STAT pathways in cytokine signaling and myeloproliferative disorders. Genes Cancer. 2010; 1: 979–993.
- Takahashi K, Patel KP, Kantarjian H, et al. JAK2 p.V617F detection and allele burden measurement in peripheral blood and bone marrow aspirates in patients with myeloproliferative neoplasms. Blood. 2013; 122: 3784–3786.
- Jaiswal S, Fontanillas P, Flannick J, et al. Age-related clonal hematopoiesis associated with adverse outcomes. N Engl J Med. 2014; 371: 2488–2498.
- Scott L. The JAK2 exon 12 nutations: a comprehensive review. Am J Hematol. 2011; 86: 668–676.
- Gold LI, Eggleton P, Sweetwyne MT, et al. Calreticulin: non-endoplasmic reticulum functions in physiology and disease. Faseb J. 2010; 24: 665–683.
- Pietra D, Rumi E, Ferretti VV, et al. Differential clinical effects of different mutation subtypes in CALR-mutant myeloproliferative neoplasms. Leukemia. 2016; 30: 431–438.
- Kaushansky K. Thrombopoietin. N Engl J Med. 1998; 339: 746–754.
- Staerk J, Lacout C, Sato T, Smith SO, Vainchenker W, Constantinescu SN. An amphipathic motif at the transmembrane-cytoplasmic junction prevetns autonomous activation of the thrombopoietin receptor. Blood. 2006; 107: 1864–1871.
- Haider M, Elala YC, Gangat N, Hanson CA, Tefferi A. MPL mutations and palpable splenomegaly are independent risk factors for fibrotic progression in essential thrombocythemia. Blood Canc J. 2016; 10: e347.
- Ding J, Komatsu H, Wakita A, et al. Familial essential thrombocythemia associated with a dominant-positive activating mutation of the c-MPL gene, which encodes for the receptor for thrombopoietin. Blood. 2004; 103: 4198–4200.
- Beer PA, Campbell PJ, Scott LM, et al. MPL mutations in myeloproliferative disorders: analysis of the PT-1 cohort. Blood. 2008; 112: 141–149.
- Milosevic Feenstra JD, Nivarthi H, Gisslinger H, et al. Whole-exome sequencing identifies novel MPL and JAK2 mutations in triple-negative myeloproliferative neoplasms. Blood. 2016; 127: 325–332.
- Harutyunyan AS, Kralovics R. Role of germline genetic factors in MPN pathogenesis. Hematol/Oncol Clin North Am. 2012; 1037–1051.
- Cabagnols X, Favale F, Pasqiuer F, et al. Presence of atypical thrombopoietin receptor (MPL) mutations in triple-negative essiental thrombocythemia patients. Blood. 2016; 127: 333–342.
- Beer PA, Jones AV, Bench AJ, et al. Clonal diversity in the myeloproliferative neoplasms: independent origins of genetically distinct clones. Br J Haematol. 2009; 144: 904–908.
- Usseglio F, Beaufils N, Calleja A, Raynaud S, Gebart J. Detection of CALR and MPL mutations in low allelic burden JAK2 V617F essential thrombocythemia. J Mol Diagn. 2017; 19: 92–98.
- Bernal M, Jiménez P, Puerta J, Ruíz-Cabello F, Jurado M. Co-mutated CALR and MPL driver genes in a patient with myeloproliferative neoplasm. Ann Hematol. 2017; 96: 1399–1401.
- Thompson ER, Nguien T, Kankanige Y, et al. Clonal independence of JAK2 and CALR or MPL mutations in comutated myeloproliferative neoplasms demonstrated by single cell DNA sequencing. Haematologica. 2021; 106: 313–315.
- Lundberg P, Karow A, Nienhold R, et al. Clonal evolution and clinical correlates of somatic mutations in myeloproliferative neoplasms. Blood. 2014; 123: 2220–2228.
- Grinfeld J, Nangalia J, Green AR. Molecular determinants of pathogenesis and clinical phenotype in myeloproliferative neoplasms. Haematologica. 2017; 102: 2–12.
- Guglielmelli P, Lasho TL, Rotunno G, et al. The number of prognostically detrimental mutations and prognosis in primary myelofibrosis: an international study of 797 patients. Leukemia. 2014; 28: 1804–1810.
- Delhommeau F, Dupont S, Della Valle V, et al. Mutation in TET2 in myeloid cancers. N Engl J Med. 2009; 360: 2289–2301.
- Stegelmann F, Bullinger L, Schlenk RF, et al. DNMT3A mutations in myeloproliferative neoplasms. Leukemia. 2011; 25: 1217–1219.
- Ortmann CA, Kent DG, Nangalia J, et al. Effect of mutation order on myeloproliferative neoplasms. N Engl J Med. 2015; 372: 601–612.
- Nangalia J, Nice FL, Wedge DC, et al. DNMT3A mutations occur early or late in patients with myeloproliferative neoplasms and mutation order influences phenotype Haematologica. 2015; 100: e438–e442.
- O’Sullivan J, Panchal A, Yap C, et al. The mutational landscape in hydroxycarbomide-resistant/intolerant essential thrombocythemia treated on the MAJIC-ET study. Hemasphere. 2018; 2: 714.
- Zhang SJ, Rampal R, Manhouri T, et al. Genetic analysis of patients with leukemic transformation of myeloproliferative neoplasms shows recurrent SRSF2 mutations that are associated with adverse outcome. Blood. 2012; 119: 4480–4485.
- Rampal R, Ahn J, Abdel-Wahab O, et al. Genomic and functional analysis of leukemic transformation of myeloproliferative neoplasms. Proc Natl Acad Sci USA. 2014; 111: E5401–E5410.
- Jutzi JS, Bogeska R, Nikoloski G, et al. MPN patients harbor recurrent truncating mutations in transcription factor NF-E2. J Exp Med. 2013; 210: 1003–1019.
- Ward AF, Braun BS, Shannon KM. Targeting oncogenic Ras signaling in hematologic malignancies. Blood. 2012; 120: 3397–3406.
- Jones AV, Chase A, Silver RT, et al. JAK2 haplotype is a major risk factor for the development of myeloproliferative neoplasms. Nat Genet. 2009; 41: 446–449.
- Hermouet S, Vilaine M. The JAK2 46/1 haplotype: a marker of inappropriate myelomonocytic response to cytokine stimulation, leading to increased risk of inflammation, myeloid neoplasm, and impaired defense against infection? Haematologica. 2011; 96: 1575–1579.
- Jäger R, Harutyunyan AS, Rumi E, et al. Common germline variation at the TERT locus contributes to familial clustering of myeloproliferative neoplasms. Am J Hematol. 2014; 89: 1107–1110.
- Tapper W, Jones AV, Kralovics R, et al. Genetic variation at MECOM, TERT, JAK2 and HBS1L-MYB predisposes to myeloproliferative neoplasms. Nat Commun. 2015; 6: 6691.
- Hinds DA, Barnolt K, Mesa RA, el al. Germ line variants predispose to both JAK2 V617F clonal hematopoiesis and myeloproliferative neoplasms. Blood. 2016; 128: 1121–1128.
- Loh PR, Genovese G, McCaroll SA. Monogenic and polygenic inheritance become instruments for clonal selection. Nature. 2020; 584: 136–141.
- Bao EL, Nandakumar SK, Liao X. Inherited myeloproliferative neoplasm risk affects haematopoietic stem cells. Nature. 2020; 586: 769–775.
- Mambet C, Babosova O, Defour JP, et al. Cooccurring JAK2 V617F and R1063H mutations increase JAK2 signaling and neutrophilia in myeloproliferative neoplasms. Blood. 2018; 132: 2695–2699.
- Lanikova L, Babosova O, Swierczek S, et al. Coexistence of gain-of-function JAK2 germ line mutations with JAK2V617F in polycythemia vera. Blood. 2016; 128: 2266–2270.
- Schulze S, Stengel R, Jaekel N, et al. Concomitant and noncanonical JAK2 and MPL mutations in JAK2V617F‐ and MPLW515 L‐positive myelofibrosis. Genes Chromos Cancer. 2019; 58: 747–755.
- Benton CB, Prajwal CB, DiNardo CD, et al. Janus Kinase 2 variants asscociated with the transformation of myeloproliferative neoplasms into acute myeloid leukemia. Cancer. 2019; 125: 1855–1866.
- Marty C, Saint‐Martin C, Pecquet C, et al. Germ‐line JAK2 mutations in the kinase domain are responsible for hereditary thrombocytosis and are resistant to JAK2 and HSP90 inhibitors. Blood. 2014; 123: 1372–1383.
- Ricci K, Huber E, Raj A, et al. Compound heterozygosity of two novel JAK2 mutations in hereditary essential thrombocythemia implicates important monomer-monomer interactions in thrombopoiesis signaling. Blood. 2016; 128: 3137.
- Kapralova K, Horvathova M, Pecquet C, et al. Cooperation of germline JAK2 mutations E846D and R1063H in hereditary erythrocytosis with megakaryocytic atypia. Blood. 2016; 128: 1418–1423.
- Srour SA, Devesa SS, Morton LM, et al. Incidence and patient survival of myeloproliferative neoplasms and myelodysplastic/myeloproliferative neoplasms in the United States, 2001-12. Br J Haematol. 2016; 174: 382–396.
- Harman D. The free radical theory af aging: effect of age on serum copper levels. J Gerontol. 1965; 20: 151–153.
- Kurosawa S, Iwama A. Aging and leukemic evolution of hematopoietic stem cells under various stress conditions. Inflamm Regen. 2020; 40: 29.
- Sørensen AL, Hasselbalch HC. Smoking and Philadelphia-negative chronic myeloproliferative neoplasms. Eur J Haematol. 2016; 97: 63–69.
- Schultz MB, Sinclair DA. When stem cells grow old: phenotypes and mechanisms of stem cell aging. Development. 2016; 143: 3–14.
- Kovtonyuk LV, Fritsch K, Feng X et al. Inflamm-aging of hematopoiesis, hematopoietic stem cells, and the bone marrow microenvironment. Front Immunol. 2016; 7: 502.
- Ho YH, Méndez-Ferrer S. Microenvironmental contributions to hematopoietic stem cell aging. Haematologica. 2020; 105: 38–46.
- Barraco D, Mora B, Guglielmelli P, et al. Gender effect on phenotype and genotype in patients with post-polycythemia vera and post-essential thrombocythemia myelofibrosis: results from the MYSEC project. Blood Cancer J. 2018; 8: 89.
- Pecquet C, Diaconu CC, Staerk J, et al. Thrombopoietin receptor down-modulation by JAK2 V617F: restoration of receptor levels by inhibitors of pathologic JAK2 signaling and of proteasomes. Blood. 2012; 119: 4625–4635.
- Parganas E, Wang D, Stravopodis D, et al. Jak2 is essential for signaling through a variety of cytokine receptors. Cell. 1998; 93: 385–395.
- Hasselbalch HC, Bjørn RnME. MPNs as inflammatory diseases: the evidence, consequences, and perspectives. Mediators Inflamm. 2015; 2015: e102476.
- Vaidya R, Gangat N, Jimma T, et al. Plasma cytokines in polycythemia vera: phenotypic correlates, prognostic relevance, and comparison with myelofibrosis. Am J Hematol. 2012; 87: 1003–1005.
- Pourcelot E, Trocme C, Mondet J, Bailly S, Toussaint B, Mossuz P. Cytokine profiles in polycythemia vera and essential thrombocythemia patients: clinical implications. Exp Hematol. 2014; 42: 360–368.
- Stetka J, Vyhlidalova P, Lanikova L, et al. Addiction to DUSP1 protects JAK2V617F-driven polycythemia vera progenitors against inflammatory stress and DNA damage, allowing chronic proliferation. Oncogene. 2019; 38: 5627–5642.
Labels
Haematology Internal medicine Clinical oncologyArticle was published in
Transfusion and Haematology Today
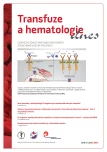
2021 Issue 3
Most read in this issue
- Laboratory diagnostics in immune-mediated thrombocytopenias
- New insights into the pathophysiology of Ph-negative myeloproliferative neoplasms
- POEMS syndrome – a rare disease associated with monoclonal gammopathy and polyneuropathy: diagnosis and treatment
- Changes in the immune system in untreated patients with chronic lymphocytic leukaemia – part 2: innate immune system