Vliv digoxinu, valproátu sodného a celekoxibu na mozkovou cyklooxygenázovou dráhu a neuron-specifickou enolázu při pentylenetetrazolem podnícené záchvaty u myší
Authors:
Vadim Tsyvunin; Sergiy Shtrygol; Mariia Mishchenko; Dmytro Lytkin; Andriy Taran; Diana Shtrygol; Tatiana Gorbach
Published in:
Čes. slov. Farm., 2023; 72, 172-183
Category:
Original Articles
doi:
https://doi.org/10.5817/CSF2023-4-172
Overview
Neuroinflammation plays an important role in the pathogenesis of epilepsy, so it is necessary to clarify the influence of standard antiepileptic drugs as well as adjuvant agents (e.g., cardiac glycoside digoxin, which previously showed a clear anticonvulsant potential) on cyclooxygenase pathway and neuron-specific enolase under the conditions of chronic epileptogenesis. The aim of the article is to determine the effect of digoxin, sodium valproate, and celecoxib per se, as well as the combination of digoxin with sodium valproate on the content of cyclooxygenase 1 and 2 types, prostaglandins E2, F2α, I2, thromboxane B2, 8-isoprostane and neuron-specific enolase in the brain of mice in the pentylenetetrazole-induced kindling model. It was found that only the combination of sodium valproate with digoxin provides a complete protective effect (absence of seizures) and shows the clearest influence on neuroinflammation markers and neuronal damage than monotherapy with each of these drugs and celecoxib, which appeared to be an ineffective anticonvulsant. The obtained results indicate that digoxin is a promising adjuvant drug to classical antiepileptic drugs (mostly sodium valproate) in epilepsy treatment.c
Keywords:
digoxin – neuron-specific enolase – valproate – cyclooxygenase – pentylenetetrazole-induced kindling – celecoxib
Introduction
According to the World Health Organization, the prevalence of epilepsy is extremely high – the incidence in the population is about 0.5–1%1). In 30 % of cases, it is impossible to achieve seizure control with standard treatments2, 3), which significantly affects patients’ quality of life and countries’ economic losses. It determines the urgency of the search for new targets of influence on epileptogenesis as well as the discovery of new combinations of anticonvulsant drugs with other medicines.
In previous studies, we found the presence of a pronounced anticonvulsant effect of digoxin per se, as well as its potentiating effect on classical commonly used antiepileptic drugs (AEDs) in acute primarily generalized models of seizure induced by pentylenetetrazole (PTZ), picrotoxin, thiosemicarbazide, strychnine, camphor and maximal electroshock in mice. It has been verified that low-dose digoxin clearly increases the antiepileptic potential of sodium valproate, carbamazepine, lamotrigine, levetiracetam, topiramate, clonazepam, and phenobarbital, supplying a pronounced pharmacological effect of their subeffective doses even under incomplete seizure control – the equivalent of drug-resistant epilepsy4–7).
As for the multifaceted epileptogenesis, it becomes even more critical when researching a new potential anticonvulsant medicine to investigate its effect on various links of epilepsy pathways. It is known that neuroinflammation plays an important role in the pathogenesis of brain diseases, including epilepsy8). Cyclooxygenase (COX) and prostaglandins (PG) are the main targets for reducing neuroinflammation. Both isoforms of COX, COX-1, and COX-2, are expressed in the CNS9). The COX-1 isoform is found mainly in microglia, while COX-2 is found mainly in neurons10). The presence of COX-2 is restricted to cell bodies and dendritic areas of neurons in the hippocampus, cerebral cortex, and amygdala11). The expression of COX-1 in the brain increases with aging, which indicates not only the homeostatic role of this enzyme12). In addition, there are studies indicating the constitutive expression of the COX-2 isoform in the brain13) and its participation in synaptic plasticity14). The fact that the COX-2 enzyme is expressed throughout the CNS and can be increased under the influence of any pathophysiological stimuli determines the researchers’ interest in studying its role in seizure development.
Besides, one of the triggering factors of neuroinflammation is oxidative stress, which initiates the widespread death of neurons. Certain damage to neurons contributes to the formation of excitatory neurotransmitters, a deficiency of macroergic substances, the accumulation of calcium currents, nitric oxide, pro-inflammatory cytokines, and other cerebral substance systems, which collectively contribute to strengthening the lipoperoxidation process15, 16). The active radicals destabilize cell membranes’ work and accelerate lipids’ degradation, contributing to the excess influx of glutamate, Ca2+ ions, and other altering components through micro-defects inside the cell17).
Isoprostanes are a group of prostaglandin isomers, while 8-isoprostane (8-iso-PGF2α) is an isomeric prostaglandin F2α. It is used as a marker of oxidative stress since the content of 8-isoprostane is directly proportional to the amount of free radicals16, 18). With a number of diseases, including neurological ones, the level of isoprostanes in biological samples increases significantly19, 20). An increase in the content of 8-isoprostane in the brain is observed under experimental seizure models, in particular, those induced by kainate21).
NSE (neuron-specific enolase) is an enzyme of the glycolytic chain, the activity of which increases significantly in case of neurons being damaged. Therefore, an increase in the level of NSE can be considered a highly specific and sensitive biochemical indicator reflecting neuronal damage22).
Therefore, the cerebral content of COX-1, COX-2, different prostaglandins, and 8-isoprostane and NSE can be considered targets for the mechanism of action of AEDs. Taking into account the previously studied antiepileptic activity of digoxin4–7) as well as the recently discovered anti-inflammatory properties of cardiac glycosides23), we wanted to investigate its effect in combination with classical AED sodium valproate on the pro-inflammatory factors in the brain of mice under the pentylenetetrazole (PTZ)-induced kindling model.
The aim of the present study is to determine the effect of digoxin in a subcardiotonic dose per se and in combination with sodium valproate as well as celecoxib per se on the content of cyclooxygenase 1 and 2 types (COX-1, COX-2), PG E2, F2α, I2, thromboxane (TX) B2, NSE and 8-isoprostane in the mice brain under the condition of the PTZ-induced kindling. This animal model of kindling and increasing the state of epileptic readiness of the brain with long-term use of subthreshold doses of a convulsant approximates the natural conditions of long-term formation of the epileptic brain24). Therefore, this model is relevant when studying the effect of drugs on various links of epileptogenesis, including the neuroinflammatory pathway.
Experimental part
Materials
Medicines were used in the form of officinal drugs: digoxin (Digoxin-Zdorovie® 0.25mg/ml solution for injection, DNCLZ/Zdorovie, Ukraine); sodium valproate (Depakine® 57.64 mg/ml syrup, Sanofi Aventis, France) and celecoxib (Celebrex® 200 mg capsules, Pfizer, USA).
The convulsive agent pentylenetetrazole (PTZ) was used as a substance (Sigma, USA). Cyclooxygenase-1(COX-1),cyclooxygenase-2(COX-2), prostaglandin E2 (РGE2), prostaglandin F2alpha (PGF2α), prostacyclin (PGI2), thromboxane B2 (TXB2), 8-isoprostane and neuron-specific enolase (NSE) species-specific enzyme immunoassay kits (MyBio-Source, USA) were used.
Methods
Study design
Control mice (groups 1 and 2 – vehicle control and positive control, respectively) received intragastrically and subcutaneously the solvent (water) in a 0.1 ml/10 g body weight for 16 days. For kindling modeling, PTZ was used in a dose of 30 mg/kg intraperitoneally25) for 16 days. All studied medicines were administered 30 min before PTZ. Digoxin was used subcutaneously at a subcardiotonic dose of 0.8 mg/kg (1/10 LD50) 4–7), which showed an anticonvulsant effect previously.
The classical AED sodium valproate was administered intragastrically at a dose of 150 mg/kg26). This dose usually does not provide the maximum protective effect, which allows for detecting both the weakening and strengthening of the anticonvulsant activity4).
Celecoxib was administered intragastrically at a dose of 4 mg/kg27). Celecoxib as a reference drug was chosen based on the assumption that COX-2 (rather than COX-1) plays a key role in neuroinflammation. Additional use of non-selective inhibitors of COX-1/2 (in particular, acetylsalicylic acid and other agents) as reference drugs were not advisable for bioethical reasons, since the main aim of the study was to determine the neurochemical mechanisms of digoxin – both per se and in combination with classical AED, but not nonsteroidal anti-inflammatory drugs.
Observation was carried out live daily, in the morning, by three identical researchers for all days. At the same time, the researchers were “blinded” as to which medicine (or combination) was administered to the experimental groups of animals before.
Observation of animals after PTZ injection lasted 1 hour. A latency period of the first seizures, number of days with convulsions, number of mice with seizures as well as seizure severity were observed. Seizure severity was evaluated using a modified Racine scale4, 28) in points where 0 means no seizures; 1 – ear and facial jerks, sporadic head nodding; 2 – clonus of one forelimb without upright position; 3 – bilateral forelimb clonus with/without rearing; 4 – bilateral foreand hindlimb clonus and falling with/without rearing; 5 – tonic extension. Days with convulsions is calculated by the number of days where at least 1 mouse with convulsions in the experimental group has been detected. This indicator, together with the daily number of mice with seizures, characterizes the degree of a protective effect of the studied drugs in the dynamics of kindling, taking into account even the most sensitive animals.
After PTZ-induced kindling, the brains of mice were examined by immunochemical methods. On the 16th day of the experiment, 1 hour after the last drug administration (in groups VС and PС – after solvent administration), animals were euthanized by dislocation of the cervical vertebrae25). The brains of 6 animals from each group were used. The brain was immediately removed and frozen with liquid nitrogen, stored in a freezer at –70 °C. Before examination, the samples were homogenized with standard phosphatebuffered saline (buffer-to-material ratio is 10 : 1) at a temperature of –4 °C and thrice centrifugated at 6000 × g for 10 min.
Experimental animals and grouping
The study was conducted on 56 outbred female albino mice weighing 20–24 g kept in a well-ventilated room on a standard vivarium diet (commercial laboratory rodent chow) with free access to food and water under controlled conditions (20–24 °C and 50% humidity with a 12 h light/dark cycle).
The animals were obtained from the Educational and Scientific Institute of Applied Pharmacy of the National University of Pharmacy (NUPh), Kharkiv, Ukraine.
The experiments were carried out in accordance with the principles and requirements of the EU Directive 2010/63/EU (2010) on the protection of animals used for scientific purposes and approved by the NUPh Bioethical Committee (Protocol No. 3, September 10, 2020).
The animals were randomly divided into 6 groups:
-
- Group 1 (n = 6): vehicle control (VC) – for neurochemical studies.
- Group 2 (n = 10): positive control (PC), PTZ-induced kindling without treatment;
- Group 3 (n = 10): PTZ-induced kindling + sodium valproate;
- Group 4 (n = 10): PTZ-induced kindling + digoxin;
- Group 5 (n = 10): PTZ-induced kindling + sodium valproate + digoxin;
- Group 6 (n = 10): PTZ-induced kindling + celecoxib.
Statistical Analysis
The results were expressed as mean ± standard error of the mean (M ± m). Statistical differences between groups were analyzed using a parametric one-way ANOVA test as well as a non-parametric Kruskal-Wallis test (for mean seizure severity score). Fisher’s angular transformation was used for the results in the alternative form (% of mice with seizures, days with convulsions, highest seizure severity). The levels of statistical significance were considered as p < 0.05, 0.01, and 0.001.
Results
Beneficial effects of digoxin and sodium valproate on the course of PTZ-induced kindling
The results of the influence of studied drugs on the PTZ-induced kindling process are shown in Figures 1–2 and Table 1.
Repeated administration of PTZ at a sub-convulsive dose (30 mg/kg) every subsequent day (for 16 days in a row) in all groups (except the valproate + digoxin group) led to the onset and gradual increase in the severity of seizures.
In the control, digoxin, and celecoxib groups, the latency period lasted three days. The first clonic seizures were observed in 1 mouse (10% of the number of animals in the group) on the 4th day of the experiment. In the sodium valproate group, the latency period made 6 days – the first paroxysms were registered in 1 mouse (10% of the number of animals in the group) on the 7th day of the convulsant administration (Fig. 1).
The total number of days marked by seizures in the control, digoxin, and celecoxib groups was 13, while under sodium valproate, the number of such days was 8 (Table 1), which was significantly lower compared to the three previous groups (p < 0.05).
However, the combination of valproate and digoxin provided a complete protective effect: there were no seizures during all 16 days of observation, which is statistically significant compared to all other experimental groups (p < 0.05). The same effect of the combination has been verified in relation to the severity of seizures – the combined use of digoxin with valproate statistically significantly (p < 0.05) reduced the highest severity score on days 10, 11, and 13 compared to the control group as well as on days 14–16 – compared to other experimental groups (Table 1).
The number of animals with seizures gradually increased in the control, sodium valproate, digoxin, and celecoxib groups. It reached a maximum on the 14th day. It was, as we can see in Figure 1, 80 % in the control group, 50% on the background of sodium valproate, and 30% under the influence of digoxin (p < 0.05 compared to the indicators of the control and celecoxib groups), 70% on against celecoxib. The absence of animals with convulsions under the valproate + digoxin combination reliably distinguished this group from all others (p < 0.05).
The effect of digoxin, sodium valproate, and, especially, a combination of these drugs (but not celecoxib) is also manifested in a decrease in the progression of the seizure severity compared to control in the dynamics of PTZ-induced kindling. At the same time, the mean seizure severity score in the digoxin and valproate groups from days 13 to 16 was statistically significantly lower (p < 0.05) than in the control and celecoxib groups (Fig. 2). The specificity of the course of the convulsive syndrome was that the clonic attack was not limited to one forelimb: in the case of onset from separate forelimb, the convulsions also covered the contralateral one. As can be seen from Table 1, the severity of seizures in the valproate group was limited to bilateral clonic convulsions of the forelimbs without loss of postural control (3 points).
In the control, digoxin, and celecoxib groups, the most severe seizures involved the hindlimbs and were accompanied by falling, corresponding to 4 points. The severe tonic extension (5 points), which usually results in the death of animals with seizure models, was not observed in any case.
Therefore, the protective effect of sodium valproate and digoxin on the PTZ-induced kindling model has certain differences. Sodium valproate doubles the latency period of the first attacks, reduces the number of days with seizures (p < 0.05), and tends to increase the number of mice with convulsions by 1.6 times. Digoxin significantly reduces the number of animals with seizures by 2.7 times (p < 0.05) but does not affect the latency period of convulsions and the number of days with seizures. However, digoxin potentiates the anticonvulsant activity of sodium valproate, which provides a full protective effect. In contrast to sodium valproate, digoxin, and their combination, celecoxib shows only a weak tendency toward anticonvulsant activity, consisting of only a slight reduction in the number of animals with seizures.
Influence on cerebral cyclooxygenase pathway
The results of the influence of studied drugs on the cyclooxygenase pathway indicators of the arachidonic acid cascade and 8-isoprostane are presented in Tables 2–3.
In the PC group, pronounced changes in the studied markers, which indicate the development of a neuroinflammatory reaction against the background of long-term PTZ administration, were observed. Compared with the indicators of the VC group, the content of COX-1 statistically significantly increased (p < 0.001) by 12.81%, while the expression of COX-2 increased by 1.64 times (p < 0.001) (Table 2), the content of PGF2α increased by 27.68% (p < 0.001), TXB2 doubled (p < 0.001), and the levels of PGE2 and PGI2 decreased by 42.33% and 49.76%, respectively (p < 0.001) (Table 3). A significant increase in the content of 8-isoprostane by 2.75 times (p < 0.001) (Table 3) also has been verified.
In the digoxin group, the level of COX-1 and COX-2 decreased by 11.99% and 59.19%, respectively (p < 0.001) (Table 2). It should be noted that the amount of COX-1 and COX-2 in the brain instead of measuring their activities has been determined based on the assumption of the nuclear impact of digoxin and its influence on enzymes’ expression. The content of prostaglandins also changed significantly (p < 0.001): PGE2 increased by 33.36%, PGF2α decreased by 17.59%, PGI2 increased by 14.94%, and TXB2 decreased almost twice (Table 3). Reduction in the intensity of oxidative stress is evidenced by a decrease in the level of 8-isoprostane by 57.72% (Table 3).
Sodium valproate shows a statistically significant (p < 0.001) influence on all investigated markers of the arachidonic acid cascade: the level of COX-1 decreased by 16.22%, COX-2 – by 52.95% (Table 2), PGE2 content increased by 61.66%, PGF2α decreased by 11.88%, PGI2 increased by 36.04%, and TXB2 decreased by 45.87% (Table 3). Sodium valproate also showed pronounced antioxidant and neurocytoprotective properties in the PTZ-kindled brain, significantly (p < 0.001) reducing the level of 8-isoprostane by 40.75% (Table 3) compared to the PC group.
In the framework of combining digoxin with sodium valproate, the expression of inflammatory enzymes was suppressed (Table 2): the level of COX-1 decreased by 14.36%, and COX-2 – by 65.78%. The concentration of PGE2 increased by 1.78 times (p < 0.001), the content of PGF2α decreased by 22.41% (p < 0.001), and PGI2 increased by 1.89 times (p < 0.001), while TXB2 decreased by 2.61 times (p < 0.001) (Table 3). The content of 8-isoprostane decreased by 2.87 times (p < 0.001) (Table 3) compared with PC. Almost all studied markers came as close as possible to the indicators of the VC under the combination use. While comparing the combination’s and sodium valproate’s effects per se, a significantly more pronounced effect on all markers, except COX-1, attracts attention. Almost all studied markers came as close as possible to the indicators of the VC under the combination use.
Despite lipophilic properties that allow the capability to penetrate through the blood-brain barrier and belonging to highly selective COX-2 inhibitors, celecoxib had almost no effect on the increased level of COX-2, which remained 1.54 times higher than VC (p < 0.001). But the increased content of COX-1 under the influence of celecoxib decreases to the level of VC, which was statistically significantly different from the level of PC (p < 0.001), the content of PGE2 increased by 50.22% (p < 0.001), PGF2α decreased by 9.75% (p < 0.01), the level of PGI2 almost did not change, and TXB2 decreased by 31.25% (p < 0.001) compared to the corresponding indicators of PC. The level of 8-isoprostane decreased by 25.72% (p < 0.001) against PC, which indicates the moderate antioxidant properties of celecoxib, for which it was significantly (p < 0.001) inferior to sodium valproate, digoxin, and especially their combination.
Influence on neuronal damage
The results of the influence of studied drugs on the content of NSE – an indicator of neuronal damage – are presented in Table 4.
Significant growth of NSE level by 17.46 times (p < 0.001) compared with theVC group in the PC animals indicates the pronounced damage to neurons under the influence of PTZ. Neurocytoprotective properties of digoxin have been verified by a decrease in the cerebral content of NSE by 65.29% (p < 0.001) compared with the PC group. Sodium valproate also significantly reduces the PTZ-induced growth of NSE level – by 50.60% (p < 0.001). The combination of digoxin with sodium valproate shows the most significant protection against neuronal damage, decreasing NSE’s content by 8.40 times (p < 0.001) compared to PC – much closer to the VC level than the drugs per se. Celecoxib practically did not show neurocytoprotective properties, as it reduced the cerebral content of NSE by only 3.20% compared with PC (p > 0.05).
Discussion
According to the experimental data, the seizure syndrome on the chronic model of epilepsy (PTZ-induced kindling), celecoxib practically did not affect the course of the experimental seizure syndrome. However, on the model of acute PTZ-induced seizures, this highly selective COX-2 inhibitor exerts an anticonvulsant effect, which is obviously related to the anti-inflammatory properties of celecoxib and explained by a facilitatory role for the COX-2/PGE2 pathway in the acute seizures, induced by PTZ27). In the case of PTZ-induced kindling, the effect of celecoxib was insufficient to reduce the severity of seizures. Instead, not only sodium valproate but also digoxin at a subcardiotonic dose significantly improve the course of the convulsive syndrome in this model of chronic epileptogenesis. At the same time, digoxin and sodium valproate, as can be seen from Figs. 1–2, statically significantly reduce the number of animals with convulsions and the severity of seizures (13–16 days). Also, digoxin significantly reduces the number of mice with seizures in the final phase of kindling (13–16 days), when this marker rapidly increases in the control group, while sodium valproate also prolongs the latency period of seizures by 2-fold, significantly reduces the total number of days with convulsions and prevents loss of postural control (Fig. 2, Table 1).
An important result of the experiment is the detection of synergism of the anticonvulsant effect of digoxin and valproate used together. This consists of the complete absence of seizures throughout the experiment. Literature data, which are confirmed by our results, show that this is largely due to the antiinflammatory properties of digoxin.
Indeed, the last decade has seen the discovery of previously unknown properties of cardiac glycosides, in particular, their effect on inflammation23). It was found that digoxin provides its anti-inflammatory activity impairing the overproduction of pro-inflammatory cytokines29). The beneficial action of ouabain has been established on lipopolysaccharides-activated astrocytes, which indicates its effectiveness in neuroinflammation23, 30). On the other hand, proven anti-inflammatory properties, including under conditions of neuroinflammation, are inherent to classical AEDs – in particular, valproates31–33). However, how significant is the role of anti-inflammatory properties of cardiac glycosides and AEDs in the anticonvulsant activity? And also, how correlated are the anti-inflammatory and anticonvulsant activity of known anti-inflammatory drugs?
Experimental and clinical studies have shown that inflammatory molecules (cytokines, chemokines, and prostaglandins) are released by the brain and endotheliocytes of cerebral capillaries, affecting the excitability and functional state of neurons34). We investigated the effect of the cardiac glycoside digoxin in a subcardiotonic dose, classic AED sodium valproate, the selective COX-2 inhibitor celecoxib per se, and the combination of digoxin with sodium valproate on the cyclooxygenase pathway of the arachidonic acid cascade, the content of 8-isoprostane and NSE in the brain of mice under PTZ-induced kindling.
The course of PTZ-induced kindling varies significantly depending on the studied drugs. Under the influence of sodium valproate, the nonantiepileptic drug digoxin, and a combination of these agents, a distinct anticonvulsant effect was observed, while celecoxib, on the contrary, almost did not improve the course of seizures in mice. Thus, in the model of chronic epileptogenesis, the highly selective COX-2 inhibitor did not show efficacy as an anticonvulsant, and the high level of 8-isoprostane and NSE in the mice brain indicates the absence of a clear protective effect on oxidative stress and neuronal destruction under long-term exposure to PTZ. Thus, although celecoxib exerts a moderate anticonvulsant impact in an acute model of PTZ-induced seizures27, 35), its protective effect is insufficient under the model of chronic epileptogenesis.
It is known that both isoforms of COX can be induced in the brain under the influence of various pathophysiological factors: convulsions, craniocerebral trauma, or the process of epileptogenesis36). The results of the study on the kindling model prove that COX-1 and COX-2 are involved in the process of epileptogenesis: foamy and branched microglial cells expressing COX-1 increase, and the content of this enzyme progressively increases37). Under normal conditions, COX-2 is expressed at extremely low basal levels in the hippocampus of mice, but the level of COX-2 begins to increase 1 h after the induction of status epilepticus and reaches a maximum within 4–24 h, almost 60 times the basal level38). In our study, PTZ provides the increase in the cerebral content of COX-2 more than 1.6 times, which was not observed against the background of the use of drugs with high anticonvulsant activity (sodium valproate, digoxin, and their combination), while the content of COX-1 increased slightly (by 1.13 times). Predominant inhibition of COX-2 by these drugs may indicate that with their long-term use, the risk of side effects due to inhibition of constitutive COX-1 will decrease.
Activation of COX-2 initiates the synthesis of all five types of prostanoids in the brain: PGE2, PGD2, PGF2α, PGI2, and TXA2, which can activate a total of nine receptors associated with G protein-coupled receptors (GPCRs), which mediates multiple deleterious and beneficial effects after prolonged seizures39, 40). Experimental evidence suggests the role of PGE2 as a key mediator in COX-2 signaling41). Moreover, administration of exogenous PGE2, but not PGD2 or PGF2α, increases seizure frequency and amplitude of excitatory postsynaptic potentials 42). In addition, PGE2 increases glutamate release from astrocytes43), suggesting a role for PGE2 in excitatory transmission in the brain. Recent experimental studies suggest that PGE2 is an important mediator of inflammation in chronic seizure syndrome through the activation of four GPCRs, namely EP1EP4, which regulate a wide range of physiological and pathological functions in the brain44). For example, the EP2 receptor is constitutively expressed in the CNS under normal conditions to regulate important physiological brain functions such as synaptic plasticity, cognition, and emotion45, 46).
In our study, in the PTZ-induced kindling model, PGE2 expression in the PC group decreases by 42%, while in the seizure-treated groups, it increases and approaches the VC level, especially under the influence of the combination valproate + digoxin. It is probably due to COX-2 suppression and reduced neuronal damage due to prolonged exposure to the convulsant, as evidenced by a marked decrease in NSE levels.
PGF2α expression is known to increase epilepsy47, 48). Experimentally, PGF2α levels begin to increase in rat brains within 10 min of subcutaneous injection of kainic acid47), possibly by conversion from PGE2 or PGD2. In an immunohistochemical study of the rat brain 30 min after kainic acid-induced seizures, PGF2α was detected in hippocampal CA3 neurons and dentate gyrus granule cells, suggesting that these populations of hippocampal neurons are the main source of PGF2α in the brain after rope seizure induction, again still, probably due to PGE2 isomerization48). Taken together, these data demonstrate that PGF2α is rapidly synthesized in response to seizure induction and is involved in the acute phase of seizure activity. It can be assumed that the effect of the activation of PGF2α receptors on the survival of neurons and the intensity of seizures depends on the duration of the seizure syndrome, the functional characteristics of tissues, and signaling pathways involved in asynchronous excitation, etc. In the context of these data, the increase in the content of PGF2α in the brain of animals of the PC group and the decrease in its level against the background of the protective effect of anticonvulsants indicate the benefit of the proconvulsant value of PGF2α in the conditions of chronic epileptogenesis. Only the combination of digoxin with sodium valproate provided a full protective effect and reduced the cerebral content of PGF2α to the level of VС, in contrast to monotherapy with these drugs, the anticonvulsant effect of which was less and was not accompanied by a complete normalization of this prostaglandin content.
The role of PGI2 in epileptogenesis is not studied sufficiently. In our study, the level of PGI2 increased statistically significantly (p < 0.001) compared to the PC under the influence of all studied groups except for celecoxib. Against the background of the use of a combination of digoxin and sodium valproate, the expression of PGI2 was most pronounced, which is evidence of a positive effect under conditions of chronic epileptogenesis.
The prostacyclin antagonist is TXB2, which stimulates platelet aggregation and causes vasoconstriction. Its elevated level is dangerous in view of the development of epileptic brain ischemia49). In our study, a twofold increase in the content of TXB2 in the brains of animals of the PC group and its decrease to an almost normal (sodium valproate), normal (digoxin), and even subnormal level (valproate + digoxin) proves the pathogenetic value of TXB2 in the mechanisms of chronic epileptogenesis and its antithromboxan effect as one of the links of the mechanism of AEDs’ neuroprotective activity. Special attention is drawn to the lowest antithromboxan effect of celecoxib, which corresponds to its insufficient effectiveness as an anticonvulsant.
Thus, chronic administration of low-dose PTZ is associated with the activation of neuroinflammation. PTZ-induced kindling is associated with an increase in the cerebral expression of COX-1 and 2 and a pronounced imbalance in the components of the cyclooxygenase pathway of the arachidonic acid cascade. At the same time, the pathogenetic role of individual prostaglandins as well as thromboxane in epileptogenesis requires further clarification. Also, the decrease in the cerebral content of PGE2 and PGI2 (but not PGF2α and TXB2) against the background of an increase in the expression of the enzymes producing them requires in-depth study. Digoxin, valproate, and especially their combination normalize the PTZ-induced changes of the COX-1/2 content (digoxin, probably due to its steroid structure, penetrates into the nucleus of glial cells and regulates the gene expression of enzymes), as well as other cyclooxygenase pathway components in the brain, which, obviously, is one of the possible mechanisms for implementing their anticonvulsant action. Interestingly, celecoxib does not cause a significant reduction of COX-2 and this entire pathway, although it is a highly selective inhibitor of this isoenzyme and penetrates into the central nervous system through its lipophilic properties27, 50). Obviously, the anti-inflammatory effect of celecoxib is limited by the absence of pronounced influence on the cerebral COX-2 isoform. The weak influence of celecoxib on neuroinflammation corresponds to the lack of anticonvulsant activity in the experiment. At the same time, the verified significant effect of digoxin and valproate (especially when used in combination) on the cyclooxygenase pathway corresponds to the previously established anti-inflammatory properties of cardiac glycosides as well as AEDs. These results suggest that the effect on neuroinflammation is one of the components of successful seizure control.
Neuroinflammation and oxidative stress are closely related phenomena that may reinforce each other51). Studies on animals have shown that inhibition of reactive oxygen species production improves survival and cognitive deficits as well as reduces neuronal damage after status epilepticus52). In the model of electrically induced epileptic status in rats, it was shown that the redox-sensitive protein HMGB1 links oxidative stress with neuroinflammation in epilepsy53). In rats with a status epilepticus, the antioxidant drug N-acetyl-cysteine, a precursor of glutathione, and sulforaphane, which activates the transcription factor NRF2, blocked the generation of reactive oxygen species and prevented the cytoplasmic translocation of HMGB1 in hippocampal neurons and glia. These effects inhibited the progression of seizures. During treatment, the drug did not affect the course of epileptic status. Still, after the withdrawal, the onset of epileptic seizures was delayed, and the frequency of chronic seizures was significantly reduced53). These data prove that oxidative stress is an important pathogenic factor in the development of convulsions. We found a 2.75-fold increase in the cerebral content of 8-isoprostane – an indicator of an oxidative stress – in the animals of the PC group and its decrease in the groups of effective anticonvulsants to full normalization under the influence of the combination of valproate + digoxin, confirming the significant role of oxidative stress in brain damage during kindling as well as the antioxidant effect of anticonvulsants as a component of their protective effect mechanism.
NSE is a biomarker associated with microglial activation, which enables the assessment of the degree of damage to brain neurons, which occurs in ischemia and various metabolic, inflammatory, and neurodegenerative diseases, including epilepsy54). As a result of brain cell damage, the level of neuro-specific enzymes and their isozymes in the extracellular environment increases. Therefore, the degree of severity of structural and functional disorders of the CNS biomembranes can be determined by the degree of NSE increase in brain tissue. Due to the destruction of brain cells, the amount of NSE in the blood increases also. In studies of ischemic strokes in adult patients, a correlation was found between increased levels of NSE in the blood and the severity of neurological deficits22).
A 17.46-fold increase in the level of NSE in the PC group compared to the VC indicator specifies serious damage of mice neurons. The combination of valproate + digoxin effectively reduced NSE concentration the most (by 8.4 times). It proves these drugs’ neuroprotective effects and correlates with their high anticonvulsant activity. Due to their pronounced anticonvulsant activity, digoxin, valproate, and their combinations apparently contribute to the reduction of neuronal damage and, accordingly, to less induction of cerebral COX-2. The underlying causes and subtle mechanisms of these differences in effects on the inflammatory pathway require further clarification. Unlike sodium valproate and digoxin, celecoxib did not affect NSE content. This corresponds to the absence of a pronounced effect on the course of PTZ-induced kindling and, together with the condition of the cyclooxygenase pathway, indicates a limitation of the anti-inflammatory and antioxidant properties of celecoxib in the CNS without its neuroprotective effect.
Along with the pharmacodynamic interaction of digoxin with sodium valproate, which probably leads to an increase in the anticonvulsant effect, the role of possible pharmacokinetic interaction cannot be excluded. The pharmacokinetic interaction of several antiepileptic drugs with digoxin is known55–57). For example, phenytoin, carbamazepine, and topiramate can decrease plasma concentrations of digoxin. However, neither digoxin nor valproate are P-glycoprotein inhibitors, which excludes this mechanism in possible CNS accumulation of drugs. Certainly, to clarify the possibility of changing the concentration of sodium valproate in the blood and, especially, in the brain under the influence of digoxin, special studies are needed, which are to be assigned to the next stage of our study.
Conclusions
The results of the study of the effect of digoxin, sodium valproate, their combination, and celecoxib on the cyclooxygenase pathway of the arachidonic acid cascade, the content of 8-isoprostane and NSE in the brain of mice under the PTZ-induced kindling are presented. Only the combination of sodium valproate with digoxin provides a complete protective effect (absence of seizures) and shows the most pronounced effect on markers of neuroinflammation and neuronal damage than monotherapy with each of these drugs and celecoxib, which appeared to be an ineffective anticonvulsant. Against the background of the combination of valproate + digoxin, the increased level of cerebral COX-1, COX-2, PGF2α, and TXB2 was most effectively reduced, while the reduced content of PGE2 and PGI2 increased. A statistically significant decrease in the level of 8-isoprostane in the valproate + digoxin group indicates a beneficial effect on the course of oxidative stress caused by long-term administration of PTZ. Distinct neuroprotective properties of the valproate + digoxin combination were revealed, as indicated by a statistically significant decrease in the elevated level of NSE in the animal brain. The obtained results indicate digoxin is a promising agent for further study as an adjuvant component to classical antiepileptic drugs (mostly sodium valproate) in epilepsy treatment.
Acknowledgments
This work is a part of the scientific project “Rationale for improving the treatment of multidrug-resistant epilepsy through the combined use of classical anticonvulsant medicines with other drugs” (No. 0120U102460, 2020/2022) supported by the Ministry of Health of Ukraine and carried out at the expense of the State Budget of Ukraine.
Conflicts of interest: none.
Vadim Tsyvunin, PhD.
ORGANOSYN LTD, 6-L Akademika Knyshova str. 03022 Kyiv, Ukraine
e-mail: tsyvunin-vad@ukr.net
S. Shtrygol’ • M. Mishchenko • A. Taran
Department of Pharmacology and Pharmacotherapy
National University of Pharmacy, Kharkiv, Ukraine
D. Lytkin
Educational and Scientific Institute of Applied Pharmacy
National University of Pharmacy, Kharkiv, Ukraine
D. Shtrygol’
Department of Clinical Neurology, Psychiatry, and Narcology
School of Medicine, V. N. Karazin
Kharkiv National University, Kharkiv, Ukraine
T. Gorbach
Department of Biological Chemistry
Kharkiv National Medical University, Kharkiv, Ukraine
Sources
- Thijs R. D., Surges R., O’Brien T. J., Sander J. W. Epilepsy in adults. Lancet 2019; 393(10172), 689–701.
- Rosillo-de la Torre A., Luna-Bárcenas G., Orozco-Suárez S., Salgado-Ceballos H., García P., Lazarowski A., Rocha L. Pharmacoresistant epilepsy and nanotechnology. Front. Biosci. (Elite Ed) 2014; 6, 329–340.
- Weaver D. F., Pohlmann-Eden B. Pharmacoresistant epilepsy: Unmet needs in solving the puzzle(s). Epilepsia 2013; 54, 80–85.
- Tsyvunin V., Shtrygol’ S., Shtrygol’ D. Digoxin enhances the effect of antiepileptic drugs with different mechanism of action in the pentylenetetrazole-induced seizures in mice. Epilepsy Res. 2020; 167, 106465.
- Tsyvunin V., Shtrygol’ S., Havrylov I., Shtrygol’ D. Lowdose digoxin enhances the anticonvulsive potential of carbamazepine and lamotrigine in chemo-induced seizures with different neurochemical mechanisms. ScienceRise, Pharm. Sci. 2021; 6(34), 58–65.
- Tsyvunin V., Shtrygol’ S., Shtrygol’ D., Mishchenko M., Kapelka I., Taran A. Digoxin potentiates the anticonvulsant effect of carbamazepine and lamotrigine against experimental seizures in mice. Thai. J. Pharm. Sci. 2021; 45(3), 165–171.
- Tsyvunin V., Shtrygol’ S., Mishchenko M., Shtrygol’ D. Digoxin at a sub-cardiotonic dose for the modulation of the anticonvulsive potential of valproate, levetiracetam, and topiramate in experimental primary generalized seizures. Ces.Slov. Farm. 2022; 71, 76–86.
- Dhir A. An update of cyclooxygenase (COX)-inhibitors in epilepsy disorders. Expert Opin. Investig. Drugs 2019; 28(2), 191–205.
- Zidar N., Odar K., Glavac D., Jerse M., Zupanc T., Stajer D. Cyclooxygenase in normal human tissues – is COX-1 really a constitutive isoform, and COX-2 an inducible isoform? J. Cell Mol. Med. 2009; 13(9B), 3753–3763.
- Hoozemans J. J., Rozemuller A. J., Janssen I., De Groot C. J., Veerhuis R., Eikelenboom P. Cyclooxygenase expression in microglia and neurons in Alzheimer’s disease and control brain. Acta Neuropathol. 2001; 101(1), 2–8.
- Wang H., Ye M., Yu L., Wang J., Guo Y., Lei W., Yang J. Hippocampal neuronal cyclooxygenase-2 downstream signaling imbalance in a rat model of chronic aluminium gluconate administration. Behav. Brain. Funct. 2015; 11, 8.
- Bosetti F., Sang-Ho Choi Rethinking the role of cyclooxygenase-1 in neuroinflammation: More than homeostasis. Cell Cycle 2010; 9(15), 2919–2920.
- Yermakova A., O’Banion M. K. Cyclooxygenases in the central nervous system: implications for treatment of neurological disorders. Curr. Pharm. Des. 2000; 6(17), 1755–1776.
- Bazan N. G. COX-2 as a multifunctional neuronal modulator. Nat Med 2001; 7(4), 414–415.
- Seo W., Oh H. Comparisons of acute physiological parameters influencing outcome in patients with traumatic brain injury and hemorrhagic stroke. Worldviews Evid Based Nurs 2009; 6(1), 36–43.
- Niizuma K., Endo H., Chan P. H. Oxidative stress and mitochondrial dysfunction as determinants of ischemic neuronal death and survival. J. Neurochem. 2009; 109(1), 133–138.
- Wu L., Xiong X., Wu X., Ye Y., Jian Z., Zhi Z., Gu L. Targeting Oxidative Stress and Inflammation to Prevent Ischemia-Reperfusion Injury. Front. Mol. Neurosc. 2020; 13, 28.
- Herasymchuk N. M. 8-isoprostane as the main marker of oxidative stress. Zaporož. Med. Ž. 2018; 6(111), 853–859.
- Czerska M., Zieliński M., Gromadzińska J. Isoprostanes – A novel major group of oxidative stress markers. Int. J. Occup. Med. Environ. Health. 2016; 29(2), 179–190.
- Miller E., Morel A., Saso L., Saluk J. Isoprostanes and Neuroprostanes as Biomarkers of Oxidative Stress in Neurodegenerative Diseases. Oxid. Med. Cell Longev. 2014; 2014, 572491.
- Patel M., Liang L. P., Roberts L. J. Enhanced hippocampal F2-isoprostane formation following kainate-induced seizures. J. Neurochem. 2001; 79, 1065–1069.
- Akcan A., Akyildiz H., Deneme M. A., Akgun H., Aritas Y. Granulomatous lobular mastitis: A complex diagnostic and therapeutic problem. World J. Surg. 2006; 30, 1403– 1409.
- Fürst R., Zündorf I., Dingermann T. New Knowledge About Old Drugs: The Anti-Inflammatory Properties of Cardiac Glycosides. Planta Med. 2017; 83(12–13), 977– 984.
- Singh T., Mishra A., Goel R. K. PTZ kindling model of epileptogenesis, refractory epilepsy, and associated comorbidities: relevance and reliability. Metab. Brain. Dis. 2021; 36(7), 1573–1590.
- Hock F. J. Drug Discovery and Evaluation: Pharmacological Assays. Springer International Publishing 2016.
- Duveau V., Pouyatos B., Bressand K., Bouyssi’eres C., Chabrol T., Roche Y., Roucard C. Differential effects of antiepileptic drugs on focal seizures in the Intrahippocampal kainate mouse model of mesial temporal lobe epilepsy. CNS Neurosci. Ther. 2016; 22, 497–506.
- Oliveira M. S., Furian A. F., Royes L. F., Fighera M. R., Fiorenza N. G., Castelli M., Machado P., Bohrer D., Veiga M., Ferreira J., Cavalheiro E. A., Mello C. F. Cyclooxygenase-2/PGE2 pathway facilitates pentylenetetrazol-induced seizures. Epilepsy Res. 2008; 79(1), 14–21.
- Racine R. J. Modification of seizure activity by electrical stimulation. II. Motor seizure. Electroencephalogr. Clin. Neurophysiol. 1972; 32, 281–294.
- Shaker M. E., Hamed M. F., Shaaban A. A. Digoxin mitigates diethylnitrosamine-induced acute liver injury in mice via limiting production of inflammatory mediators. Saudi Pharm. J. 2022; 30(3), 291–299.
- Kinoshita P. F., Yshii L. M., Vasconcelos A. R., Orellana A. M., Lima L., Davel A. P., Rossoni L. V., Kawamoto E. M., Scavone C. Signaling function of Na,K-ATPase induced by ouabain against LPS as an inflammation model in hippocampus. J. Neuroinflammation 2014; 11, 218.
- Chen J. Y., Chu L. W., Cheng K. I., Hsieh S. L., Juan Y. S., Wu B. N. Valproate reduces neuroinflammation and neuronal death in a rat chronic constriction injury model. Sci. Rep. 2018; 8(1), 16457.
- Itoh K., Taniguchi R., Matsuo T., Oguro A., Vogel C., Yamazaki T., Ishihara Y. Suppressive effects of levetiracetam on neuroinflammation and phagocytic microglia: A comparative study of levetiracetam, valproate and carbamazepine. Neurosci. Lett. 2019; 708, 134363.
- Raza M., Dhariwal M. A., Ageel A. M., Qureshi S. Evaluation of the anti-inflammatory activity of sodium valproate in rats and mice. Gen. Pharmacol. 1996; 27(8), 1395–1400.
- Vezzani A., Viviani B. Neuromodulatory properties of inflammatory cytokines and their impact on neuronal excitability. Neuropharmacology 2015; 96, 70–82.
- Mishchenko M., Shtrygol’ S., Lozynskyi A., Khomyak S., Novikov V., Karpenko O., Holota S., Lesyk R. Evaluation of anticonvulsant activity of dual COX-2/5-LOX inhibitor darbufelon and its novel analogues. Sci. Pharm. 2021; 89(2), 22.
- Dash P. K., Mach S. A., Moore A. N. Regional expression and role of cyclooxygenase-2 following experimental traumatic brain injury. J. Neurotrauma 2000; 17(1), 69–81.
- Tanaka S., Nakamura T., Sumitani K., Takahashi F., Konishi R., Itano T., Miyamoto O. Stageand region-specific cyclooxygenase expression and effects of a selective COX-1 inhibitor in the mouse amygdala kindling model. Neurosci. Res. 2009; 65(1), 79–87.
- Jiang J., Yang M. S., Quan Y., Gueorguieva P., Ganesh T., Dingledine R. Therapeutic window for cyclooxygenase-2 related anti-inflammatory therapy after status epilepticus. Neurobiol Dis 2015; 76, 126–136.
- Dey A., Kang X., Qiu J., Du Y., Jiang J. Anti-inflammatory small molecules to treat seizures and epilepsy: From bench to bedside. Trends Pharmacol. Sci. 2016; 37, 463–484.
- Yu Y., Nguyen D. T., Jiang J. G protein-coupled receptors in acquired epilepsy: Druggability and translatability. Prog. Neurobiol. 2019; 183, 101682.
- Sang N., Zhang J., Marcheselli V., Bazan N.G., Chen C. Postsynaptically synthesized prostaglandin E2 (PGE2) modulates hippocampal synaptic transmission via a presynaptic PGE2 EP2 receptor. J. Neurosci. 2005; 25, 9858–9870.
- Chen C., Bazan N. G. Endogenous PGE2 regulates membrane excitability and synaptic transmission in hippocampal CA1 pyramidal neurons. J. Neurophysiol. 2005; 93, 929–941.
- Bezzi P., Carmignoto G., Pasti L., Vesce S., Rossi D., Rizzini B. L., Pozzan T., Volterra A. Prostaglandins stimulate calcium-dependent glutamate release in astrocytes. Nature 1998; 391, 281–285.
- Nagib M. M., Yu Y., Jiang J. Targeting prostaglandin receptor EP2 for adjunctive treatment of status epilepticus. Pharmacol. Ther. 2020; 209, 107504.
- Savonenko A., Muñoz P., Melnikova T., Wang Q., Liang X., Breyer R. M., Andreasson K. Impaired cognition, sensorimotor gating, and hippocampal long-term depression in mice lacking the prostaglandin E2 EP2 receptor. Exp. Neurol. 2009; 217, 63–73.
- Yang H., Zhang J., Breyer R. M., Chen C. Altered hippocampal long-term synaptic plasticity in mice deficient in the PGE2 EP2 receptor. J. Neurochem. 2009; 108, 295– 304.
- Baran H., Heldt R., Hertting G. Increased prostaglandin formation in rat brain following systemic application of kainic acid. Brain Res. 1987; 404(1–2), 107–112.
- Takei S., Hasegawa-Ishii S., Uekawa A., Chiba Y., Umegaki H., Hosokawa M., Woodward D. F., Watanabe K., Shimada A. Immunohistochemical demonstration of increased prostaglandin F(2)alpha levels in the rat hippocampus following kainic acid-induced seizures. Neuroscience 2012; 218, 295–304.
- Steinhauer H. B., Anhut H., Hertting G. The synthesis of prostaglandins and thromboxane in the mouse brain in vivo. Influence of drug induced convulsions, hypoxia and the anticonvulsants trimethadione and diazepam. Naunyn Schmiedeberg’s Arch. Pharmacol. 1979; 310(1), 53–58.
- Moghimipour E., Salami A., Monjezi M. Formulation and Evaluation of Liposomes for Transdermal Delivery of Celecoxib. Jundishapur J. Nat. Pharm. Prod. 2015; 10(1), e17653.
- Lugrin J., Rosenblatt-Velin N., Parapanov R., Liaudet L. The role of oxidative stress during inflammatory processes. Biol. Chem. 2014; 395(2), 203–230.
- Pearson J. N., Rowley S., Liang L. P., White A. M., Day B. J., Patel M. Reactive oxygen species mediate cognitive deficits in experimental temporal lobe epilepsy. Neurobiol. Dis. 2015; 82, 289–297.
- Pauletti A., Terrone G., Shekh-Ahmad T., Salamone A., Ravizza T., Rizzi M., Pastore A., Pascente R., Liang L. P., Villa B. R., Balosso S., Abramov A. Y., van Vliet E. A., Del Giudice E., Aronica E., Patel M., Walker M. C., Vezzani A. Targeting oxidative stress improves disease outcomes in a rat model of acquired epilepsy. Brain 2019; 142(7), e39.
- Mu R. Z., Liu S., Liang K. G., Jiang D., Huang Y. J. A Meta-Analysis of Neuron-Specific Enolase Levels in Cerebrospinal Fluid and Serum in Children with Epilepsy. Front. Mol. Neurosci. 2020; 13, 24.
- Johannessen S. I., Landmark C. J. Antiepileptic drug interactions – principles and clinical implications. Curr. Neuropharmacol. 2010; 8(3), 254–267.
- Patsalos P. N., Fröscher W., Pisani F., van Rijn C. M. The importance of drug interactions in epilepsy therapy. Epilepsia 2002; 43(4), 365–385.
- Perucca E. Clinically relevant drug interactions with antiepileptic drugs. Br. J. Clin. Pharmacol. 2006; 61(3), 246–255.
Labels
Pharmacy Clinical pharmacologyArticle was published in
Czech and Slovak Pharmacy
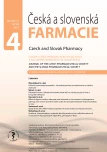
2023 Issue 4
Most read in this issue
- Celkové intravenózne anestetiká – farmakodynamika, farmakokinetika a chirálne vlastnosti
- Studium protizánětlivých vlastností hustého extraktu Tribulus terrestris L.
- Vliv digoxinu, valproátu sodného a celekoxibu na mozkovou cyklooxygenázovou dráhu a neuron-specifickou enolázu při pentylenetetrazolem podnícené záchvaty u myší
- Diazotační titrace v lékopisné kontrole jakosti léčiv a návrhy pro jejich revizi v Evropském lékopisu*