Agcu bimetallic nanoparticles modified by polyvinyl alcohol - the cells viability study in vitro
Authors:
Vladan Bernard 1; Ondřej Zobač 2; Marcela Vlková 3; Vojtěch Mornstein 1; Jiří Sopoušek 2
Authors place of work:
Department of Biophysics, Faculty of Medicine, Masaryk University, Brno, Czech Republic
1; Department of Chemistry, Faculty of Science, Masaryk University, Brno, Czech Republic
2; Department of Clinical Immunology and Allergy, St Anne’s Faculty Hospital and Faculty of Medicine, Masaryk University, Brno, Czech Republic
3
Published in the journal:
Lékař a technika - Clinician and Technology No. 1, 2018, 48, 5-10
Category:
Původní práce
Summary
The effects of elementary metallic nanoparticles on living objects as cytotoxicity or antibacterial activity are widely known. Ag nanoparticles are a suitable and well known example. Nanoparticles formed by an alloy of Ag and Cu stabilized by polyvinyl alcohol were examined on two human tumor cells - ovarian carcinoma cells A2780 and skin melanoma cells A375. Bimetallic AgCu nanoparticles were synthesized by using a method of chemical co-reduction of silver nitrate and copper (II) nitrate hydrate. The nanoparticles were characterized by electron microscopy and by measurement of zeta potential. Cell viability was tested by using an MTT (tetrazole colorimetric viability assay) test. The effect on cell apoptosis and necrosis was measured by using flow cytometry. The experimental results indicate a differentiated impact of nanoparticles on the cells used. A more significant effect of viability decrease was observed for A2780 cells. The cell death caused by the nanoparticles used was observed particularly in the form of initial and advanced apoptosis for both cells lines, necrosis was observed to a lesser extent. The synthesized bimetallic nanoparticles seem to be a suitable candidate for targeted suppression of cell proliferation.
Keywords:
AgCu NPs, cells A2780, cells A375, nanoparticle, viability
Introduction
The amount of metallic nanoparticles (NPs) in the environment and in everyday consumer products is increasing rapidly. NPs are included, for example, in cosmetics, clothing, personal care products, and even many foods items [1]. For this reason, there are legitimate concerns about the possible interaction between NPs and living tissues. The toxicity of some of the particles was demonstrated in both lower and higher organisms [2–4]. Silver, copper or titanium oxide NPs are among the frequently mentioned NPs exhibiting cell toxicity and an antifungal/antibacterial effect, for example [5–7]. It is logical that NPs, mainly metallic, are considered to be one of the most promising agents for cancer therapy. They have been discovered to be drug carriers, photothermal agents, contrast agents, radiosensitisers or they have direct effect on cells viability [8, 9].
These biological properties of NPs can be observed because of their extremely small dimensions as well as their shape, crystalline structure, composition, and surface structure.
Bimetallic NPs are also increasingly synthesized, but still not to such a great extent as monometallic NPs. Bimetallic NPs represent combinations of various metallic materials formed into objects at sizes below 100 nm. One of the possible combinations of metallic materials is silver and copper, formed into AgCu NPs with various surface modifications [10]. AgCu nanomaterials find application in chemistry as a catalyst [11] or in electrochemistry [12]. AgCu NPs find applications in medicine too. The addition of AgCu NPs improves corrosion resistance of amalgam compo-sition in dentistry [13]. AgCu bimetallic particles
exhibit toxicity and a manifest antibacterial effect [14], which is even much higher than the effect of Ag NPs alone [15]. Despite all these facts, AgCu NPs are not often presented and described in biomedical applications. We assume that the research area of AgCu NPs is still open in this respect.
The aim of this study was to design, produce and test bimetallic AgCu NPs with the purpose to affect tumor cell viability. The structure of NPs and types of metallic materials were chosen based on the individual characteristics and effects of the Ag and Cu. As already mentioned, AgCu NPs composites have better antimicrobial properties compared to the Ag or Cu NPs alone [14]. There was an effort to shift the research of NPs’ effects from the antibacterial to the cytotoxic level. Tested AgCu NPs were modified by polyvinyl alcohol (PVA), which ensures their shape and size stability in time without aggregation – the stability of NPs was observed over a time period of more than three months after synthesis. The PVA surfactant used was selected after previous tests with other surfactants. All other tested surfactants showed more significant toxic properties compared to PVA on carcinoma cells [16]. The use of PVA as a stabilization component of NPs is known, especially in connection with Ag NPs [17, 18], but the reported toxicity was tested just on the bacterial level. The aim of the present study was to clarify effect on tumor cells in vitro.
Materials and methods
Cell cultures and chemicals
Human ovarian carcinoma cell line A2780 and human skin melanoma cell line A375 were used, obtained from the European Cell Culture Collection. Roswell Park Memorial Institute medium (RPMI medium - 1640) with L-glutamine (Bio Tech, Ltd., Prague, Czech Republic) supplemented with 10% fetal calf serum (Bio Tech, Prague, Czech Republic) and 100 μg∙ml-1 streptomycin/penicillin (Bio Tech, Prague, Czech Republic) was used. The cell line was grown in cell culture flasks in an atmosphere of 95% air and 5% CO2 at 37 °C. The cells were detached from the glass by trypsin addition (Bio Tech, Prague, Czech Republic).
AgCu nanoparticle
The samples of the AgCu nanoalloy colloids were synthesized by chemical coeduction of silver nitrate and copper (II) nitrate hydrate as precursors and with polyvinyl alcohol (PVA) as a surfactant and sodium borohydride as reducing agent in methanol p.a. at 40 °C under a nitrogen atmosphere. The metal precursor amounts were equivalent to a particular stoichiometric ratio Ag – 28.1 wt% Cu in a eutectic alloy. Synthesized nanoparticles were separated by centrifugation for 10 min at 6000 rpm. The nanoparticles were washed to remove organic-soluble residues by three or more cycles of resuspending in deionized Millipore water. The Ag and Cu metal content was analyzed by inductivelycoupled plasma optical emission spectroscopy (ICP-OES, iCAP 6500 Duo Thermo, UK) calibrated on Ag and Cu pure metals. The hydro-dynamic size of AgCu NPs in the colloidal state was measured by the dynamic light scattering (DLS) method on a Zetasizer Nano ZS ZEN 3500 instrument (Malvern Instruments, UK) [19]. Zeta potential was also measured by DIP-cell on the same instrument. The metal cores of the AgCu NPs were investigated by transmission electron microscopy (TEM) because this technique is insensitive to elements of the organic shell. The size and shape of AgCu NP cores were investigated using a CM12 STEM microscope (Philips).
Viability test
The following procedure was used to compare the viability of cells A2780 and A375 incubated with AgCu NPs with a control group of cells: a cell’s suspension was obtained by trypsinization of cells adhering to the flask bottom. To the well of a 96-well plate containing cells in concentration of 5∙104 ml-1 in RPMI medium, a calculated volume of AgCu NPs stock solution was added to achieve a final concentration of nanoparticles of 1, 2, 4, 6, 8, 10 and 50 mg∙l-1. An equal volume of phosphate-buffered saline (PBS) free of NPs was added to the control cells. No trypsin was added. After incubation for 48 hours, the cells were washed in PBS and evaluated by a standard mitochondrial MTT test of viability [20]. Using an EL800 microplate reader (Bio-Tek, USA) the absorbance of a color product in each well was recorded at 570 nm. The absorbance value for NPs alone was subtracted from all corresponding cells’ samples. The amount of the color product is directly proportional to the metabolic activity of mitochondria (i.e., viability) in living cells.
The action of PVA alone was also tested. The solution of PVA in concentration equal to the concentration of PVA in samples of NPs of concentration 5 mg∙l-1 and 50 mg∙l-1 was added into a cell suspension of the 5∙104 cell in RPMI medium and seated on 96-well plate. The incubation time was 48 hours. The viability was tested by using an MTT test. The absorbance value was recorded at 570 nm.
Flow-cytometric analyses
The early apoptosis, late apoptosis, necrosis and normal cell population were detected by using Annexin V- Dyomics 647 (Exbio, Czech Republic) and Propidium Iodide (PI) (Sigma Aldrich, USA). The measurements were carried out on the unaffected cells control sample and on cells incubated with NPs of concentration 5 mg∙l-1 (close to IC50 - the half maximal inhibitory concentration) at a time of 48 hours. The labeling process was as follows: cells were washed with PBS, centrifugated at 1000 rpm, fixed by 500 μl Annexin V Binding Buffer (Exbio, Czech Republic) per sample and stained with 5 μl Annexin V Dyomics 647 per sample for 30 min on ice and 5 μl PI per sample. The amount of cells per one sample of flow-cytometric analysis was 1∙106.
Samples were acquired using a Navios flow cytometer (10 colors, 3 lasers) (Beckman Coulter, USA) and cytometry data (LMD files) were analyzed with Kaluza software (Beckman Coulter, Inc, USA).
Statistical analysis
The absorbance value for each sample was converted into cell viability as follows: the median absorbance value of the control group “contr” was taken as 100%; the absorbance of each other sample was expressed as a percentage of the control value, i.e., its viability relative to that of the control sample. Because of a nonnormal distribution of the values for individual groups, the non-parametric Mann-Whitney t-test at a significance level of p = 0.05 was used. Samples with corresponding concentration of NPs were compared with each other. The statistical software STATISTICA 12 (StatSoft, Inc., USA) was used to calculate the median and the upper and lower quartiles.
The data of viability of each experimental sample shown in boxplot graphs were obtained from 8 different analyses.
Results
NPs characteristic
The size and shape of AgCu NPs colloids were observed by TEM. The TEM images obtained showed nanoparticles of size below 100 nm, as is documented in Figure 1. Observed nanoparticles have a regular spherical shape, without major shape deviations.
The average diameter of NPs obtained by using the DLS method was 64.04±9.6 nm. The percentage of individual components of NPs was also measured overall composition of the sample was 64.5 wt% for Ag and 35.5 wt% for Cu. The mass of the organic layer of PVA of the dried nanoparticles was about 90%. Nanoparticles alone represented only 10% of the mass. Zeta potential measured for AgCu NPs with an organic layer formed by PVA was -16.4 mV. The results from basic characterization of AgCu NPs are shown in Table 1.
Viability test
The effect of AgCu NPs and PVA alone on viability of cells A2780 and A375 were tested. The viability values of cells in the experimental samples with different final concentrations of NPs (1, 2, 4, 6, 8, 10 and 50 mg∙l-1) were compared with the value of the control group (contr). The results of this testing for both cell lines used (A2780 and A375) are presented in Figure 2. The presented box-plots graphs show that the effect of AgCu on cell viability is different for different cell lines. A more significant effect was observed for ovarian carcinoma cell lines A2780 compared with melanoma skin cells A375. The IC50 value for A2780 cells was defined as 6–6.5 mg∙l-1 in comparison to IC50 values for A375 cells in a range of 35–40 mg∙l-1 (with regard to the variance of data). It is evident that for a similar viability effect it is necessary to increase the concentration of NPs for skin melanoma cells. The significant differences between A2780 and A375 cell viability were not observed for lower concentrations of NPs; significant differences were found at concentrations higher than 4 mg∙l-1 for all used cells (Table 2).
We made an attempt to determine the influence of PVA on decreases in cell viability. The PVA alone was tested using both cell lines. PVA was applied in a concentration equal to the concentration of PVA in NPs samples of concentration of 5 mg∙l-1 and 50 mg∙l-1. The results show only a relatively weak influence of this surfactant on cell viability in comparison of effect of NPs. A decrease of cells’ viability to the value of 76% was observed for concentrations of 50 mg∙l-1 for cell line A2780 and 90% in case of cell line A375 (Figure 3).Symbols: * statistical significance at level p < 0.05, + no statistical significance at level p < 0.05
Flow-cytometric analyses
Flow-cytometric analyses were carried out in order to determine the state of the cell suspension after exposure to NPs. The percentage of normal cells, early apoptotic cells, late apoptotic cells and necrotic cells was measured. The results obtained showed differences in effects of NP samples for both cell lines in comparison with control (Figure 4). A large number of cells was observed in the stage of apoptosis, 78.5% (59.0% when control was subtracted) for cell line A2780 and 83.2% (79.4% when control was subtracted) for cell line A375. The amounts of necrotic cells were observed as 1.7% for A375 cells compared to a value of 0.2% for cell line A2780.
Discussion
We used two different types of tumor cells lines, ovarian carcinoma cells A2780 and skin melanoma cells A375. It is substantiated by different sensitivity of various cell lines to applied nanoparticles generally [21]. This phenomenon was observed in our study as well.
It is clear from the data obtained that the synthesized AgCu/PVA NPs exhibited an ability to reduce cell viability. The toxicity of NPs was higher for the ovarian carcinoma cell line A2780 in comparison with A375 cells, as was documented by using MTT testing of cell viability. Based on the use of PVA without NPs (control groups), we can say that the major effect is conditioned by the presence of NPs. The IC50 value for A2780 cells was measured in a range of 6–6.5 mg∙l-1, IC50 value for A375 cells was 35–40 mg∙l-1. We can compare the observed IC50 dose of AgCu NPs with results of other authors (obtained by MTT test, only for pure Ag and Cu NPs). The silver dose for IC50 was documented as 19.33 mg∙l-1 for THP-I cells [4] or by other authors as 35.6 mg∙l-1 for HaCaT cells [22]. The lower concentrations were observed for commercial Ag NPs [21], where the IC50 were 2.7 mg∙l-1 for SVK14 cells, and 1.9 mg∙l-1 for NIH3T3 cells. The IC50 was 15 mg∙l-1 for Cu NPs, and 50 mg∙l-1 for CuO NPs [23]. The various sizes of NPs were used in all the studies. It is also one of the factors determining the final toxicity effect. The effect of newly synthesized AgCu/PVA NPs seems to be adequate to other results of other authors. The beneficial long stability was reached thanks to PVA modification.
The value of Zeta potential of studied AgCu NPs can be compared with other authors. The AgCu NPs stabilized by sapota and latex of DLS size 40 nm showed Zeta value of -15.24 mV [24]. In this case, the size and namely Zeta potential are almost the same. The same authors show the AgCu NPs with DLS size 25 nm, where the Zeta potential was -10.22 nm. The paper from Patra and Dasgupta commented that neither size nor zeta potential alone determine the optimal cellular response induced by NPs [25], but the effect of charge of NPs on affinity to cells was documented [26].
The observed main type of cell death for both cell lines after being affected by AgCu/PVa was apoptosis. This result is consistent with the observation of the previous study on Ag NPs [21]. However, this is in contrast with some other studies with Cu or CuO NPs that show a higher incidence of necrosis [23]. The mechanism leading to apoptosis death can be multiple, with Reactive oxygen species being one of the intermediate steps in this action. Other described factors caused by NPs are DNA damage, membrane leakage, and inhibition of mitochondria function, membrane lipid peroxidation, decreasing of actin cytoskeletal integrity or by release of metallic ions in aqueous state [27]. It is obvious that the mechanism of cell death depends not only on the type of nanoparticles used but on the cell line too. The main cause of a difference in the effect of NPs is found mainly as the influence of cell phenotype, growth rate, metabolic rate, different ability of phagocytosis, difference in transmembrane protein [28].
Conclusion
The results of our experiments show the possibility of synthesis of AgCu NPs and their surface modification by PVA. The experiments presented showed the relatively longlasting stability and toxicity of nanoparticles determined by a selected type of metal composition on carcinoma cells. The experiments didn´t show considerable toxicity of PVA alone. It was found that the decrease of cell viability caused by presence of nanoparticles depended also on the used type of carcinoma cells. The maximal effect on viability was observed for the ovarian carcinoma cell line. The mechanism of cell death for both cells lines was determined as apoptosis. According to the results obtained, the synthesized nanoparticles may be applicable in the treatment of tumors, not just as an antibacterial agent which is relatively well known.
Acknowledgement
This project was financially supported by grant of Ministry of Education of the Czech Republic (Project COST MP0903 Action (LD 11046) and CEITEC-MU (CZ.1.05/1.1.00/02.0068). This work was also supported by Czech Ministry of Health grants NT13271-4/2012.
Vladan Bernard, Ph.D.
Department of Biophysics
Faculty of Medicine
Masaryk University
Kamenice 3, 625 00 Brno
Czech Republic
E-mail: vbernard@med.muni.cz
Phone: +420 549 496 577
Zdroje
- Weir, A., Westerhoff, P., Fabricius, L., Hristovski, K., von Goetz, N.: Titanium dioxide nanoparticles in food and personal care products, Environ Sci Technol., 2012, 46, 2242–2250.
- Kumar, A., Pandey, A. K., Singh, S. S., Shanker, R., Dhawan, A.: Cellular uptake and mutagenic potential of metal oxide nanoparticles in bacterial cells, Chemosphere, 2011, 83, 1124–1132.
- Matranga, V., Corsi I.: Toxic effects of engineered nano-particles in the marine environment: Model organisms and molecular approaches, Marine Environmental Research, 2012, 32–40.
- Lanone, S., Rogerieux, F., Geys Dupont, J., Maillot-Marechal, E., Boczkowski, J., Lacroix, G., Hoet, P.: Comparative toxicity of 24 manufactured nanoparticles in human alveolar epithelial and macrophage cell lines, Particle and Fibre Toxicology, 2009, 6, 14.
- Shin, K., Kim, D. H., Yeo, S. Ch., Lee, H. M.: Structural stability of AgCu bimetallic nanoparticles and their application as a catalyst: A DFT study, Catalysis Today, 2012, 185, 1, 94–98.
- Sha, B. Y., Gao, W., Wang, S. Q., Xu, F., Lu, T. J.: Cyto-toxicity of titanium dioxide nanoparticles differs in four liver cells from human and rat, Composites Part B: Engineering, 2011, 42, 8, 2136–2144.
- Ramyadevi, J., Jeyasubramanian, K., Marikani, A., Rajakumar, G., Rahuman, A. A.: Synthesis and antimicrobial activity of copper nanoparticles, Mater. Lett., 2012, 71, 114–116.
- Pérez-Herrero, E, Fernández-Medarde, A.: Advanced targeted therapies in cancer: Drug nanocarriers, the future of chemo-therapy, Eur J Pharm Biopharm., 2015, 93, 72–79.
- Wicki, A., Witzigmann, D., Balasubramanian, V., Huwyler, J.: Nanomedicine in cancer therapy: Challenges, opportunities, and clinical applications, Journal of Controlled Release, 2015, 200, 138–157.
- Sopoušek, J., Pinkas, J., Brož, P., Buršík, J., Vykoukal, V., Škoda, D., Stýskalík, A., Zobač, O., Vřešťál, J., Hrdlička, A., Šimbera, J.: Ag-Cu Colloid Synthesis: Bimetallic Nanoparticle Characterisation and Thermal Treatment, Journal of Nano-materials, 2014, 36, 13.
- Singh, R. P., Ramarao, P.: Cellular uptake, intracellular trafficking and cytotoxicity of silver nanoparticles, Toxicology Letters, 2012, 213, 2, 249–259.
- Easow, J. S., Selvaraju, T.: Unzipped catalytic activity of copper in realizing bimetallic Ag@Cu nanowires as a better amperometric H2O2, Electrochimica Acta, 2013, 112, 648–654.
- Chung, K. H., Hsiao, L. Y., Lin, Y. S., Duh, J. G.: Morphology and electrochemical behavior of Ag–Cu nanoparticle-doped amalgams, Acta Biomaterialia, 2008, 4, 3, 717–724.
- Kar, S., Baqchi, B., Bhandary, S., Basu, S., Nandy, P., Das, S.: Synthesis and characterization of Cu/Ag nanoparticle loaded mullite nanocomposite system: A potential candidate for antimicrobial and therapeutic applications, Biochimica and Biophysica Acta, 2014, 1840, 3264–3276.
- Taner, M., Sayar, N., Yulugb, I. G., Suzer, S.: Synthesis, characterization and antibacterial investigation of silver–copper nanoalloys, J. Mater. Chem., 2011, 21, 13150.
- Bernard, V., Zobač, O., Sopoušek, J., Mornstein, V.: AgCu Bimetallic nanoparticle under effect of low intensity ultrasound: the cell viability study in vitro, Journal of Cancer Research, 2014, 36, 13.
- Becaro, A. A., Jonsson, C. M., Puti, F. C., Siqueira, M. C., Mattoso, L. H. C., Correa, D. S., Ferreira, M. D.: Toxicity of PVA-stabilized silver nanoparticles to algae and micro-crustaceans, Environmental Nanotechnology, Monitoring & Management, 2015, 3, 22–29.
- Ali, I. O.: Synthesis and characterization of Ag0/PVA nano-particles via photo- and chemical reduction methods for anti-bacterial study, Colloids and Surfaces A: Physicochemical and Engineering Aspects, 2013, 436, 922–929.
- Sourav Bhattacharjee: DLS and zeta potential – What they are and what they are not?, Journal of Controlled Release, 2016, 235, 337–351.
- Mosmann, T.: Rapid colorimetric assay for cellular growth and survival: application to proliferation and cytotoxicity assays. J. Immunol. Methods, 1983, 16, 55–63.
- Tomankova, K., Horakova, J., Harvanova, M., Malina, L., Sou-kupova, J., Hradilova, S., Kejlova, K., Malohlava, J., Licman, L., Dvorakova, M., Jirova, D., Kolarova, H., Cytotoxicity, cell uptake and microscopic analysis of titanium dioxide and silver nanoparticles in vitro, Food and Chemical Toxicology, 2015, 85, 20–30.
- Mukherjee, S. G., O’Claonadh, N., Casey, A., Chambers, G.: Comparative in vitro cytotoxicity study of silver nanoparticle on two mammalian cell lines, Toxicology in Vitro, 2012, 26, 238–251.
- Rodhe, Y., Skoglund, S., Wallinder, I. O., Potácová, Z., Möller, L.: Show more Copper-based nanoparticles induce high toxicity in leukemic HL60 cells. Toxicol. in Vitro, 2015, 29, 1711–1719.
- Thakore, S. I. et al.: Sapota fruit latex mediated synthesis of Ag, Cu mono and bimetallic nanoparticles and their in vitro toxicity studies. Arabian Journal of Chemistry (2015), http://dx.doi.org/10.1016/j.arabjc.2014.12.042
- Patra, H. K., Dasgupta, A.: Cancer cell response to nano-particles: criticality and optimality, Nanomed. Nanotechnol. 2011, 8, 115–121.
- Alkilany, A. M., Murphy, C. J.: Toxicity and cellular uptake of gold nanoparticles: what we have learned so far? J. Nanopart. Res, 2010, 12, 2313–2333.
- Ahameda, M., AlSalhia, M. S., Siddiquib, M. K. J.: Silver nano-particle applications and human health, Clinica Chimica Acta, 2010, 411, 1841–1848.
- El-Hussein, A., Mfouo-Tynga, I., Abdel-Harith, M., Abrahamse, H.: Comparative study between the photodynamic ability of gold and silver nanoparticles in mediating cell death in breast and lung cancer cell lines. J Photochem Photobiol B., 2015, 153, 67–75.
Štítky
BiomedicínaČlánek vyšel v časopise
Lékař a technika
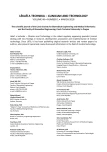
2018 Číslo 1
Nejčtenější v tomto čísle
- Median method for determining cortical brain activity in a near infrared spectroscopy image
- A comparsion of the quality of dental crowns from TI-6AL-4V and cocr alloys made with SLM technology
- Dimensionality reduction methods for biomedical data
- Patient´s respiratory curve synchronization by visual feedback application