INTRAOPERATIVE OPTICAL COHERENCE TOMOGRAPHY –AVAILABLE TECHNOLOGIES AND POSSIBILITIES OF USE. A REVIEW
Authors:
T. Benda 1; P. Studený 1,2
Authors place of work:
Oční centrum Somich, Karlovy Vary
1; Oční klinika Fakultní nemocnice Královské Vinohrady a 3. LF UK, Praha
2
Published in the journal:
Čes. a slov. Oftal., 78, 2022, No. 6, p. 277-286
Category:
Přehledový článek
doi:
https://doi.org/10.31348/2022/2
Summary
Optical coherence tomography (OCT) is a non-contact and non-invasive imaging and diagnostic method, that allows the imaging of ocular tissues on transverse sections in extremely high quality of micrometer resolution. The physical principle of OCT is analogous to ultrasound, but it uses infrared radiation instead of acoustic waves. By using a low coherent radiation source, it is possible to achieve a higher resolution. Based on the obtained data, the computer can reconstruct two or three-dimensional images of the examined tissue.
In recent years, we have seen a rapid development in ophthalmic surgery, especially in surgical instruments and imaging methods. However, the technology of surgical microscopes does not change significantly and thus becomes a limiting factor in the development of ophthalmic microsurgery.
The integration of the OCT into surgical microscopes, so the introduction of the Intraoperative Optical Coherence Tomography (iOCT), opens up further possibilities for displaying/looking at the operating field and adapting surgical techniques to the current situation during the surgery. On the contrary, the disadvantage is the prolongation of the surgery time and thus the theoretical increase in possible complications related to the surgery.
iOCT can be used for operations on the anterior and posterior segment of the eye. In anterior segment surgery, it is used mostly in penetrating and lamellar keratoplasty, glaucoma surgery and cataract surgery. In posterior segment surgery, it is used during pars plana vitrectomy.
The aim of the article is to provide a comprehensive overview of the current possibilities of using iOCT in eye surgery.
Keywords:
intraoperative OCT
INTRODUCTION
Optical coherence tomography (OCT) is a non-contact and non-invasive imaging and diagnostic method, that allows the imaging of ocular tissues on transverse sections in extremely high quality at micrometer resolution.
The introduction of OCT into clinical practice by Carl Zeiss Meditec (Dublin, CA) in 1995 changed the possibilities for examining patients with retinal diseases and became an integrated part of the work in ophthalmology practice. It enabled a better and more detailed understanding of the anatomical conditions on the retina and the pathophysiology of various retinal diseases, as well as the planning of their surgical treatment.
Gradual miniaturization of OCT into the form of portable devices and their subsequent integration into operating microscopes offers the possibility of using this imaging technology during the operation and adapting the surgical procedure to the current situation.
Intraoperative OCT (iOCT) is used for operations of the anterior and posterior segments of the eye. In anterior segment surgery, it can be used in a wide range of procedures, especially in penetrating, lamellar and endothelial keratoplasty [1–12], glaucoma surgery [13], cataract surgery [1,14–16] and tissue biopsies [1,17–18]. In posterior segment surgery, it is used during pars plana vitrectomy (PPV) to treat vitreous and retinal pathologies.
EVOLUTION OF IOCT
Only spectral OCTs are used in operating theaters for their higher speed and higher resolution. In spectral domain OCT (SD-OCT), the spectrum of all light rays at different frequencies is detected by an interferometer with a high-speed spectrometer, and signal processing is performed in the frequency domain using a Fourier transformation. The measuring beam is used very efficiently, all layers of the tissue reflecting light simultaneously so that the reference mirror does not have to move, unlike time-domain OCT (TD-OCT). SD-OCT devices thus reach a speed of 20,000–100,000 A-scans per second with an axial resolution of 5–7 μm, and hence the examination is a hundred times faster than with TD-OCT [19].
The history of using iOCT began in 2007 with the introduction of handheld SD-OCT (Bioptigen, Inc., Research Triangle Park, NC), which operated at 840 nm and produced retinal images with an axial resolution of less than 5 μm. This OCT device enabled the examination of supine adult and pediatric patients in the operating theater [20].
When using manual OCT in the operating theater, it was necessary to interrupt the surgery. This not only prolonged the surgery time, but also increased the risk of contamination of the surgery field. Attaching the handheld OCT to a microscope (microscope-mounted iOCT – MM-iOCT) improved but did not eliminate these practical problems. However, the time required to take an image of the retina has been reduced, the quality and reproducibility of the images have been improved, and OCT control using the microscope pedal has been simplified [21–23].
Although the MM-iOCT facilitated the focus of the OCT during scanning, it was still not possible to visualize the interaction of surgical instruments and operated tissue. This was made possible by the advent of the OCT integrated into the microscope (microscope-integrated iOCT – Mi-iOCT).
Mi-iOCT has built-in OCT optic path within the optics of the microscope, allowing better focusing of the scanning beam and imaging of parafocal and coaxial OCT images along with the surgical view through the microscope oculars. With Mi-iOCT, the surgeon has a real-time view of the operated tissue during the surgical manoeuvre being performed [24].
The first article on the use of Mi-iOCT in vitreoretinal surgery was published by Toth in 2010. It described his experience with a research OCT prototype integrated into a standard surgery microscope. Other early prototypes included the Cirrus SD-OCT (Carl Zeiss Meditec) integrated into a Zeiss OPMI VISU 200 surgical microscope, and the EnFocus OCT (Leica Microsystems) [24].
Currently, 3 systems are commercially available. The first is the Zeiss RESCAN 700 (Carl Zeiss Meditec), which is built into the Zeiss Lumera 700 microscope. The RESCAN 700 is controlled by the microscope pedal and iOCT data are displayed in real time in the microscope oculars and on the external monitor. The second system is iOCT (OPMedT GmbH) built into the microscope HS Hi-R NEO 900A NIR (Haag-Streit Surgical). This device also projects the iOCT image into the oculars and on the external monitor. The third system is EnFocus OCT (Leica Microsystems), which is connected to a Leica Proveo 8 surgical microscope. EnFocus has an increased number of scanning patterns for better imaging of pathological tissue and displays OCT images on the external monitor [24].
PUBLISHED ARTICLES
Since the introduction of OCT technology in operating theaters, a number of papers have been published that have dealt with this topic. However, they were relatively small and retrospective, and they corresponded in time to the stage of development of iOCT used at that time. They focused on the real feasibility (ability to take a usable OCT image during surgery) and utility of iOCT (getting an overview of tissues and/or influencing the surgical procedure based on the iOCT image). The first prospective study was the PIONEER study, which used a manual MM-iOCT, SD-OCT (Bioptigen, Inc., Research Triangle Park, NC) [9,25]. In current times it is the up-to-date Mi-iOCT which has been addressed in the prospective study DISCOVER [3,5,26–29].
The older PIONEER study (Prospective Intraoperative and Perioperative Ophthalmic ImagiNg with Optical CoherEncE TomogRaphy) from 2014, was a 2-year prospective study (single-center, multi-surgeon, consecutive, case series) that evaluated the feasibility, safety and utility of iOCT. A total of 531 operated eyes were included in the study (275 anterior segment and 256 posterior segment surgeries). OCT images were taken in the operating theatre by a trained technician. In contrast to the DISCOVER study, a manual MM-iOCT, SD-OCT (Bioptigen, Inc., Research Triangle Park, NC), was used for scanning. The stabilizing arm was specially developed for Leica and Zeiss microscopes and was compatible with the Oculus BIOM indirect imaging system as well as the contact wide-angle lens. Through the stabilization arm, the surgeon operated the OCT using the X-Y-Z pedal of the microscope, while taking images. At the end of the surgery, the surgeon recorded the imaging specifications (scan type, size, orientation, density) and answered questions about the feasibility and utility of the OCT during the surgery.
The DISCOVER study (Determination of Feasibility of Intraoperative Spectral Domain Microscope Combined/ Integrated OCT Visualization During En Face Retinal and Ophthalmic Surgery) from 2018 was a 3-year prospective study (single-site, multi-surgeon, consecutive, case series) dealing with the feasibility and utility of iOCT during both anterior and posterior segment surgeries. A total of 837 operated eyes were included in the study (244 anterior segment and 593 posterior segment surgeries). 3 prototypes of Mi-iOCT systems (Zeiss Rescan 700, Leica EnFocus and Cole Eye iOCT) were used in this study. Mi-iOCT was controlled by the surgeon and used in defined parts of the surgery. At the end of the surgery the surgeon filled in a questionnaire evaluating the feasibility and utility of Mi-iOCT.
Both studies evaluated the feasibility and utility of iOCT during ocular surgery. In the PIONEER study, images were obtained in 98% of cases (518 eyes out of 531). The surgeon identified it as useful in 48% (69 of 144) of posterior lamellar keratoplasties and in 43% (63 of 146) of PPVs with epiretinal membrane peeling (ERM). The most common anterior segment surgery was DSAEK (Descemet Stripping Automated Endothelial Keratoplasty) (n = 138) and the most common posterior segment surgery was PPV with ERM peeling (n = 154). The average time to obtain an iOCT image was 4.9 minutes. No side effects of iOCT were reported during the study.
In the DISCOVER study, images were also obtained in 98% of cases (821 eyes out of 837). Surgeons rated the Mi-iOCT information as useful in 43.4% (106 of 244) for anterior segment surgery and 29.2% (173 of 593) for posterior segment surgery. In 19% of PPVs with ERM peeling, the Mi-iOCT image did not correspond to the surgeon's original impression, when looking at the operating field through microscope optics. The most common anterior segment surgeries were DSAEK (n = 123) and DMEK (Descemet Membrane Endothelial Keratoplasty) (n = 60), and the most common posterior segment surgeries were PPV with ERM peeling (n = 121), PPV in proliferative diabetic retinopathy (n = 132), rhegmatogenic retinal detachment (n = 120), and macular hole (n = 77). No disruption of the surgery was noted during the anterior segment operations. In the case of rear segment operations, Mi-iOCT disrupted and delayed the operation in 6% of cases (n = 37), the most common reason being a failure of the microscope software with the need to restart the system. Despite the prolongation of the surgery, no side effects were reported.
CURRENT POSSIBILITIES OF USING MI-IOCT
Although a randomized study has not yet been reported, the published works describe the use of iOCT in anterior and posterior segment surgeries.
Anterior segment
Posterior lamellar keratoplasty
iOCT enables evaluation of the attachment and orientation of the transplanted lamella to the corneal stroma and provides information about residual fluid between them (Figure 1,2). This is an advantage especially in cases with worsened corneal transparency [30].
Perforating keratoplasty
iOCT helps to adapt the edges of the transplanted cornea (Figure 3).
Corneal biopsy and DALK (Deep Anterior Lamellar Keratoplasty)
iOCT gives information about the depth of trepanation in the corneal stroma [30].
Cataract surgery
iOCT may be useful in evaluation of the morphology of the corneal incision (Figure 4,5), the condition of the posterior capsule, and the position of the lens in the bag. In the study of anterior chamber depth and posterior capsule position, Fram et al found, that the iOCT-based calculation of the dioptric strength of the implanted intraocular lens was more accurate than the preoperative biometric calculation [31].
Glaucoma surgery
Additional information from iOCT may be useful in visualizing the ocular anatomy and confirming surgical goals during the surgery, such as scleral flap depth imaging (Figure 6), iris prolapse detection, and correct intubation placement (Figure 7,8) [13].
Posterior segment
iOCT is beneficial in macular surgery, retinal detachment surgery and diabetic retinopathy surgery. It is not very suitable for displaying the peripheral retina, but approximately 2/3 of the retinal surface can be displayed. Ehlers et al recommend performing the initial steps of the surgery, then using iOCT to evaluate whether the desired goal has been achieved, and then to complete the procedure, if necessary [30].
Epiretinal membrane
iOCT provides information on whether all membranes have been completely removed from the retinal surface or whether further membrane staining and peeling is required. It can also be checked whether or not the neuroretinal tissue was damaged during the procedure (Figure 9).
Vitreomacular traction
iOCT enables evaluation of the strength of vitreomacular traction in real time and visualizes thin-walled cysts, which need to be protected against rupture during vitreous separation and membrane peeling. Their perioperative evaluation and the adaptation of surgical technique affect postoperative anatomical and functional outcomes [32].
Macular hole
The anatomical configuration of the macular hole, its size and shape, affect the postoperative closure of the hole and the functional result of the surgery (Figure 10) [24]. Ehlers et al studied the dynamics of internal limiting membrane (MLI) peeling and architectural changes in the outer layers of the retina and retinal pigment epithelium using iOCT. They found that the accumulation of subretinal fluid after MLI peeling prolongs postoperative visual acuity regeneration. This perioperative iOCT finding can influence not only the MLI peeling technique itself, but also the decision whether to use an air or gas tamponade at the end of the surgery, or to aspirate the subretinal fluid and the contents of the hole with a Charles cannula [25,33].
iOCT can be also used in the case of macular hole reoperation, when the surgeon decides to transplant the free MLI piece from another part of the retina. Using iOCT, it is possible to check the exact position of the MLI in the macular hole.
Retinal detachment
Using iOCT, residual subretinal fluid in the macula can be imaged, and the surgeon's decision can then be made as to whether further aspiration of the subretinal fluid is required via a posterior retinotomy or whether postoperative face down positioning is necessary to prevent macular folds.
Diabetic retinopathy
iOCT is useful in the case of complicated findings in diabetic retinopathy, where it is necessary to identify the level of segmentation and delamination of the posterior vitreous and epiretinal membranes from the neurosensory retina, especially with impaired visibility and bleeding.
Biopsy of the retina and choroid
iOCT facilitates finding the optimal place to perform a biopsy [30].
Subretinal implants and gene therapy
In the clinics dealing with this issue, iOCT is highly valued for the ability to verify the correct placement of the retinal implant or genetic material in the subretinal space. In such surgeries, the use of iOCT is considered as a standard part of the procedure [30].
iOCT as a teaching method
Imaging of the surgery field with iOCT helps in training less experienced retinal surgeons. They can verify that their assessment of the current situation on the retina corresponds to the reality (Figure 11). They can evaluate epiretinal membranes, strength of vitreomacular traction, reaction of a macular hole to MLI peeling and aspiration of its contents, location of macular hemorrhage (Figure 12), etc.
DISCUSSION
iOCT has been in clinical use since 2007. One of the reasons why it has not yet become more widespread is the fact that many devices have actually been functional prototypes over time. The introduction of current Mi-iOCT systems represents a realistically available and usable solution. We have been using Mi-iOCT in our clinic since 2020, especially during posterior lamellar keratoplasties and pars plana vitrectomies. We use the Zeiss RESCAN 700 (Carl Zeiss Meditec) built into the Zeiss Lumera 700 microscope.
The main disadvantage that prevents more widespread expansion of iOCT is the relatively high purchase price of the devices. Surgeons see and understand the benefits of integrated OCT but must also consider the cost. More studies are needed to demonstrate the clinical benefit of iOCT and hopefully to help convince healthcare payers to consider the use of iOCT during surgery in their financial assessment of procedures.
However, it must be said that even current systems are not yet perfect and that there is room for improvements. Although the image quality is well usable, it still lags significantly behind the quality of images from separate devices commonly used in ophthalmology clinics. An improvement in quality could be achieved by increasing the intensity of the radiation used, but this must not exceed the phototoxicity limit for the retina (according to ANSI – American National Standards Institute, the current maximum exposure limit is 700 μW of continuous radiation in the 800–900 nm spectrum through a 7 mm pupil for 8 hours). It is also necessary to take into account the toxicity of the tissue dyes used during the surgery and the additional effect of microscope and endoilluminator light. Thus, the improvement in the quality of OCT imaging consists mainly in the reduction of signal loss when passing through the optical system of a microscope [20].
Mi-iOCT also lacks the eye-tracking to keep the image focused to the point of interest during eye movements during the surgery. This would significantly improve real-time imaging during the tissue manipulation. Currently it is usually necessary to interrupt the procedure to take a picture [20].
The availability of iOCT-compatible instrumentation is also related to real-time scanning. Currently-used metal tools cast a shadow on the tissues below them, making it impossible to display them (Figure 13). It is significantly better with instruments made of polyamide and silicone. Such tools are under development [20].
The ability to display iOCTs in the assistant's eyepieces would also be useful, especially for teaching. Current systems display iOCTs only in the surgeon's main eyepieces. At the same time, the image quality of the iOCT in the eyepieces lags behind the image quality on the external monitor.
Software analysis of the surgery field, which would, for example, display areas with residual membrane or assess the amount of residual subretinal fluid or fluid between the transplanted corneal lamella and stroma, would increase the utility of iOCT [30].
The use of Mi-iOCT has probably only one relative disadvantage, and that is a slight prolongation of the surgery time (approximately 60–90 s to obtain 1 image) and thus a theoretical increase in possible complications related to surgery (bleeding, infection, patient-subjective problems – pain, restlessness, poorer cooperation, and the resulting other possible risks, such as unexpected patient movement). On the other hand, the resulting operation time can be shortened, because we can assure ourselves that the manoeuvre is completed, and other unnecessary surgical steps can be avoided.
CONCLUSION
Based on published works and our practical experience, iOCT is a promising and useful addition to a standard surgery microscope. During the operation, it offers the advantage of a new view of the surgery field, allows more precise performance of the procedure, and can also change the surgeon's decisions during the surgery.
The relative disadvantage is the price of the device. However, it can be assumed that further expansion of this technology will lead to lower prices and Mi-iOCT will become standard equipment of surgery microscopes in the near future.
Despite the relatively high number of smaller retrospective studies and 2 large prospective studies, additional prospective, multicenter iOCT studies will be needed to demonstrate the clinical benefit of iOCT technology.
The authors declare that the origin and topic of the article and its publication are not in conflict of interest and are not supported by any pharmaceutical company. The work has not been assigned to another journal or published elsewhere.
Received: 30 May 2021
Accepted: 22 November 2021
Available on-line: 28 January 2022
MUDr. Tomáš Benda, FEBO
Oční centrum Somich, Karlovy Vary
Závodní 391/96C
360 06 Karlovy Vary
E-mail: tomas.benda@post.cz
Zdroje
1. Gulkas S, Cekic O. Intraoperative Optical Coherence Tomography, Novel Diagnostic Methods in Ophthalmology, IntechOpen. 2019.
2. Ehlers JP, Dupps WJ, Kaiser PK, et al. The prospective intraoperative and perioperative ophthalmic Imaging with optical coherence tomography (PIONEER) study: 2-year results. American Journal of Ophthalmology. 2014;158:999-1007.
3. Ehlers JP, Goshe J, Dupps WJ, et al. Determination of feasibility and utility of microscope-integrated optical coherence tomography during ophthalmic surgery: The DISCOVER study RESCAN results. JAMA Ophthalmology. 2015;133:1124-1132.
4. Steven P, Le Blanc C, Velten K, et al. Optimizing descemet membrane endothelial keratoplasty using intraoperative optical coherence tomography. JAMA Ophthalmology. 2013;131:1135-1142.
5. Ehlers JP, Modi YS, Pecen PE, et al. The DISCOVER study 3-year results: Feasibility and usefulness of microscope-integrated intraoperative OCT during ophthalmic surgery. Ophthalmology. 2018;125:1014-1027.
6. El-Haddad MT, Tao YK. Automated stereo vision instrument tracking for intraoperative OCT guided anterior segment ophthalmic surgical maneuvers. Biomedical Optics Express. 2015;6:3014- 3031.
7. Eguchi H, Kusaka S, Arimura-Koike E, et al. Intraoperative optical coherence tomography (RESCAN((R)) (700) for detecting iris incarceration and iridocorneal adhesion during keratoplasty. International Ophthalmology. 2017;37:761-765.
8. Titiyal JS, Kaur M, Falera R. Intraoperative optical coherence tomography in anterior segment surgeries. Indian Journal of Ophthalmology. 2017;65:116-121.
9. Au J, Goshe J, Dupps WJ Jr, Srivastava SK, Ehlers JP. Intraoperative optical coherence tomography for enhanced depth visualization in deep anterior lamellar keratoplasty from the PIONEER study. Cornea. 2015;34:1039-1043.
10. Steven P, Le Blanc C, Lankenau E, et al. Optimising deep anterior lamellar keratoplasty (DALK) using intraoperative online optical coherence tomography (iOCT). The British Journal of Ophthalmology. 2014;98:900-904.
11. Kobayashi A, Yokogawa H, Mori N, Sugyiama K. Visualization of precut DSAEK and pre-stripped DMEK donor corneas by intraoperative optical coherence tomography using the RESCAN 700. BMC Ophthalmology. 2016;16:135.
12. Saad A, Guilbert E, Grise-Dulac A, Sabatier P, Gatinel D. Intraoperative OCT-assisted DMEK: 14 consecutive cases. Cornea. 2015;34:802-807.
13. Kumar RS, Jariwala MU, Sathidevi AV, et al. A pilot study on feasibility and effectiveness of intraoperative spectral-domain optical coherence tomography in glaucoma procedures. Transl Vis Sci Technol 2015;4:2:2.
14. Hirnschall N, Amir-Asgari S, Maedel S, Findl O. Predicting the postoperative intraocular lens position using continuous intraoperative optical coherence tomography measurements. Investigative Ophthalmology & Visual Science. 2013;54:5196-5203.
15. Das S, Kummelil MK, Kharbanda V, et al. Microscope integrated intraoperative spectral domain optical coherence tomography for cataract surgery: Uses and applications. Current Eye Research. 2016;41:643-652.
16. Almutlak MA, Aloniazan T, May W. Real-time optical coherence tomography incorporated in the operating microscope during cataract surgery. Middle East African Journal of Ophthalmology. 2017;24:156-158.
17. Ruland K, Bouldin TW, Davis RM, Fleischman D. Intraoperative optical coherence tomography-assisted retrocorneal fibrous membrane biopsy and excision. American Journal of Ophthalmology Case Reports. 2018;11:101-104.
18. Siebelmann S, Horstmann J, Scholz P, et al. Intraoperative changes in corneal structure during excimer laser phototherapeutic keratectomy (PTK) assessed by intraoperative optical coherence tomography. Graefe’s Archive for Clinical and Experimental Ophthalmology. 2018;256:575-581.
19. Němec P, Kousal B, Löfflerová B. Optická koherenční tomografie-Klinický atlas sítnicových patologií. 2. vydání. Praha: Mladá fronta; 2017. 405.
20. Hahn P, Migacz J, O’Connell R, Maldonado RS, Izatt JA, Toth CA. The Use of Optical Coherence Tomography in Intraoperative Ophthalmic Imaging. Ophthalmic Surg Lasers Imaging. 2011 Jul; 42(0): S85-S94.
21. Ray R, Barañano DE, Fortun JA, et al. Intraoperative microscope- -mounted spectral domain optical coherence tomography for evaluation of retinal anatomy during macular surgery. Ophthalmology. 2011;118(11):2212-2217.
22. Binder S, Falkner-Radler CI, Hauger C, Matz H, Glittenberg C. Feasibility of intrasurgical spectral-domain optical coherence tomography. Retina. 2011;31(7):1332-1336.
23. Ehlers JP, Tao YK, Farsiu S, Maldonado R, Izatt JA, Toth CA. Visualization of real-time intraoperative maneuvers with a microscope- -mounted spectral domain optical coherence tomography system. Retina. 2013;33(1):232-236.
24. Schechet SA, Komati R, Blair MP, Hariprasad SM. Update on Intraoperative OCT for Vitreoretinal Surgery, Retinal Physician, Volume: 17, Issue: Jan/Feb 2020, page(s): 34-36, 39, 40.
25. Ehlers JP, Itoh Y, Xu LT, Kaiser PK, Singh RP, Srivastava SK. Factors associated with persistent subfoveal fluid and complete macular hole closure in the PIONEER study. Invest Ophthalmol Vis Sci. 2014;56(2):1141-1146.
26. Ehlers JP, Kaiser PK, Srivastava SK. Intraoperative optical coherence tomography using the RESCAN 700: Preliminary results from the DISCOVER study. The British Journal of Ophthalmology. 2014;98:1329-1332.
27. Runkle A, Srivastava SK, Ehlers JP. Microscope-integrated OCT feasibility and utility with the EnFocus system in the DISCOVER study. Ophthalmic Surg Lasers Imaging Retina. 2017;48(3):216- 222.
28. Ehlers JP, Kaiser PK, Srivastava SK. Intraoperative optical coherence tomography using the RESCAN 700: Preliminary results from the DISCOVER study. Br J Ophthalmol. 2014;98(10):1329-1332.
29. Ehlers JP, Goshe J, Dupps WJ, et al. Determination of feasibility and utility of microscope-integrated optical coherence tomography during ophthalmic surgery: the DISCOVER study RESCAN results. JAMA Ophthalmol. 2015;133(10):1124-1132.
30. Stuart A, Ehlers JP, Lauer AK, MacLaren RE. Intraoperative OCT: An Emerging Technology. EyeNet Magazine August 2018.
31. Fram NR, Masket S, Wang L. Comparison of intraoperative aberrometry, OCT-based IOL formula, Haigis-l, and Masket formulae for IOL power calculation after laser vision correction. Ophthalmology 2015;122:6:1096-1101.
32. Ehlers JP, Tam T, Kaiser PK, Martin DF, Smith GM, Srivastava SK. Utility of intraoperative optical coherence tomography during vitrectomy surgery for vitreomacular traction syndrome. Retina. 2014;34(7):1341-1346.
33. Ehlers JP, Xu D, Kaiser PK, Singh RP, Srivastava SK. Intrasurgical dynamics of macular hole surgery: an assessment of surgery-induced ultrastructural alterations with intraoperative optical coherence tomography. Retina. 2014;34(2):213-221.
Štítky
OftalmologieČlánek vyšel v časopise
Česká a slovenská oftalmologie
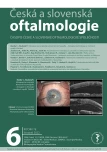
2022 Číslo 6
- Off-label užití očních kapek s obsahem bromfenaku v terapii cystoidního makulárního edému na jediném vidoucím oku u komplikované pacientky – kazuistika
- Diagnostický algoritmus při podezření na syndrom periodické horečky
- Stillova choroba: vzácné a závažné systémové onemocnění
- Kontaktní dermatitida očních víček
- Familiární středomořská horečka
Nejčtenější v tomto čísle
- LASER VITREOLYSIS IN PATIENTS WITH SYMPTOMATIC VITREOUS FLOATERS
- INTRAOPERATIVE OPTICAL COHERENCE TOMOGRAPHY –AVAILABLE TECHNOLOGIES AND POSSIBILITIES OF USE. A REVIEW
- TUBULOINTERSTITIAL NEPHRITIS WITH UVEITIS (TINU SYNDROME). A CASE REPORT
- ASSESSMENT OF CORNEAL ENDOTHELIAL LAYER IN CONTACT LENS WEARERS WITH THE AID OF AN ENDOTHELIAL MICROSCOPE