Advances in research of DNA damage and repair in cells exposed to various types of ionizing radiation in the era of super-resolution optical microscopy
Authors:
Martin Falk 1; Michael Hausmann 2
Authors‘ workplace:
Biofyzikální ústav AV ČR, v. v. i., Brno
1; Kirchhoffův institut pro fyziku, Univerzita Heidelberg
2
Published in:
Čas. Lék. čes. 2020; 159: 286-297
Category:
Review Article
Overview
The present work introduces new findings about the influence of different radiation types on the cells, with the concern on the micro- and nanodosimetric aspects of chromatin damage. Emphasized is the relationship between the physical parameters of the incident radiation (g-rays, protons and high-LET heavy ions), character of chromatin damage, ability of cells to repair and survive DNA damage, and risk of genetic changes. While confirming a positive correlation between the LET of ionizing radiation, complexity of induced DNA double-strand breaks (DSB), and biological effectiveness (RBE) of radiation, at the same time, we show that our understanding of this relationship is only incomplete. Our discovery that various accelerated ions with similar LET can damage DNA in different ways and kill cells with unequal efficiency, could serve as an example. In addition, many aspects of DSB repair remain to be explained, for instance, how the cell activates the particular repair pathway at sites of individual DSBs, and how it depends on the radiation used and the chromatin architecture.
The discussed results may be important, above all, for newly developing hadron therapy and in the context of manned interstellar flights planning. From the methodological point of view, we point to a tremendous progress in the field of optical microscopy and its research applications. In more detail, we introduce single-molecule localization microscopy (SMLM).
Keywords:
ionizing radiation of different types – DNA radiation damaging and repair – ionizing radiation-induced foci (IRIF) – chromatin architecture – super-resolution microscopy – single-molecule localization microscopy (SMLM).
Sources
- Falk M, Hausmann M, Lukášová E et al. Determining omics spatiotemporal dimensions using exciting new nanoscopy techniques to assess complex cell responses to DNA damage: part A – radiomics. Crit Rev Eukaryot Gene Expr 2014; 24: 205–223.
- Falk M, Hausmann M, Lukášová E et al. Determining omics spatiotemporal dimensions using exciting new nanoscopy techniques to assess complex cell responses to DNA damage: part B – structuromics. Crit Rev Eukaryot Gene Expr 2014; 24: 225–247.
- Bobkova E, Depeš D, Lee JH et al. Recruitment of 53BP1 proteins for DNA repair and persistence of repair clusters differ for cell types as detected by single molecule localization microscopy. Int J Mol Sci 2018; 19: 3713.
- Falk M, Lukášová E, Kozubek S. Higher-order chromatin structure in DSB induction, repair and misrepair. Mutat Res 2010; 704: 88–100.
- Pujals S, Albertazzi L. Super-resolution microscopy for nanomedicine research. ACS Nano 2019; 13: 9707–9712.
- Requejo-Isidro J. Fluorescence nanoscopy. Methods and applications. J Chem Biol 2013; 6: 97–120.
- Birk UJ. Super-resolution microscopy of chromatin. Genes (Basel) 2019; 10: 493.
- Hausmann M, Ilić N, Pilarczyk G et al. Challenges for super-resolution localization microscopy and biomolecular fluorescent nano-probing in cancer research. Int J Mol Sci 2017; 18: 2066.
- Konishi T, Kobayashi A, Tengku Ahmad TF, Wang J. Enhanced DNA double strand break repair triggered by microbeam irradiation induced cytoplasmic damage. J Radiat Cancer Res 2018; 9: 183.
- Pagáčová E, Štefančíková L, Schmidt-Kaler F et al. Challenges and contradictions of metal nano-particle applications for radio-sensitivity enhancement in cancer therapy. Int J Mol Sci 2019; 20: 558.
- Štefančíková L, Lacombe S, Salado D et al. Effect of gadolinium-based nanoparticles on nuclear DNA damage and repair in glioblastoma tumor cells. J Nanobiotech 2016; 14: 63.
- Rogakou EP, Pilch DR, Orr AH et al. DNA double-stranded breaks induce histone H2AX phosphorylation on serine 139. J Biol Chem 1998; 273: 5858–5868.
- Turinetto V, Giachino C. Survey and summary multiple facets of histone variant H2AX: a DNA double-strand-break marker with several biological functions. Nucleic Acids Res 2015; 43: 2489–2498.
- Hofer M, Falk M, Komůrková D et al. Two new faces of amifostine: protector from DNA damage in normal cells and inhibitor of DNA repair in cancer cells. J Med Chem 2016; 59: 3003–3017.
- Pejchal J, Österreicher J, Vávrová J. Biodozimetrie: dicentrická analýza a předčasná chromozomová kondenzace (PCC). Vojenské zdravotnické listy 2007; 76: 95–104.
- Markova E, Vasilyev S, Belyaev I. 53BP1 foci as a marker of tumor cell radiosensitivity. Neoplasma 2015; 62: 770–776.
- Falk M, Horáková Z, Svobodová M et al. γH2AX/53BP1 foci as a potential pre-treatment marker of HNSCC tumors radiosensitivity – preliminary methodological study and discussion. Eur Phys J D 2017; 71: 241.
- Falk M, Lukášová E, Štefančíková L et al. Heterochromatinization associated with cell differentiation as a model to study DNA double strand break induction and repair in the context of higher-order chromatin structure. Appl Radiat Isot 2014; 83 Pt B: 177–185.
- Bártová E, Kozubek S, Jirsová P et al. Nuclear structure and gene activity in human differentiated cells. J Struct Biol 2002; 139: 76–89.
- Bach M, Savini C, Krufczik M et al. Super-resolution localization microscopy of γ-H2AX and heterochromatin after folate deficiency. Int J Mol Sci 2017; 18: 1726.
- Depeš D, Lee JH, Bobkova E et al. Single-molecule localization microscopy as a promising tool for γH2AX/53BP1 foci exploration. Eur Phys J D 2018; 72: 158.
- Falk M, Falková I. Účinky ionizujícího záření na subcelulární a celulární úrovni, mechanizmy reparace DNA. In: Havránková R (ed.). Klinická radiobiologie. Grada, Praha, 2020: 67–102.
- Falk M, Falková I, Kopečná O et al. Chromatin architecture changes and DNA replication fork collapse are critical features in cryopreserved cells that are differentially controlled by cryoprotectants. Sci Rep 2018; 8: 14694.
- Rittich B, Španová A, Falk M et al. Cleavage of double stranded plasmid DNA by lanthanide complexes. J Chromatogr B Analyt Technol Biomed Life Sci 2004; 800(1–2): 169–173.
- Kousholt AN, Menzel T, Sørensen CS. Pathways for genome integrity in G2 phase of the cell cycle. Biomolecules 2012; 2: 579–607.
- Jalan M, Olsen KS, Powell SN. Emerging roles of RAD52 in genome maintenance. Cancers 2019; 11: 1038.
- Serrano L, Liang L, Chang Y et al. Homologous recombination conserves DNA Sequence integrity throughout the cell cycle in embryonic stem cells. Stem Cells Dev 2011; 20: 363–374.
- Shrivastav M, De Haro LP, Nickoloff JA. Regulation of DNA double-strand break repair pathway choice. Cell Res 2008; 18: 134–147.
- Lukášová E, Kořistek Z, Klabusay M et al. Granulocyte maturation determines ability to release chromatin NETs and loss of DNA damage response; these properties are absent in immature AML granulocytes. Biochim Biophys Acta 2013; 1833: 767–779.
- Ma J, Setton J, Lee NY et al. The therapeutic significance of mutational signatures from DNA repair deficiency in cancer. Nat Commun 2018; 9: 3292.
- Falk M, Hausmann M. A paradigm revolution or just better resolution – will newly emerging superresolution techniques identify chromatin architecture as a key factor in radiation-induced DNA damage and repair regulation? Cancers 2021; 13: 18.
- Shibata A, Conrad S, Birraux J et al. Factors determining DNA double-strand break repair pathway choice in G2 phase: DSB repair pathway choice in G2 phase. EMBO J 2011; 30: 1079–1092.
- Kakarougkas A, Jeggo PA. DNA DSB repair pathway choice: an orchestrated handover mechanism. Br J Radiol 2014; 87: 20130685.
- Amaral N, Ryu T, Li X, Chiolo I. Nuclear dynamics of heterochromatin repair. Trends Genet 2017; 33: 86–100.
- Hausmann M, Neitzel C, Bobkova E et al. Single molecule localization microscopy analyses of DNA-repair foci and clusters detected along particle damage tracks. Front Phys Sect Med Phys Imaging 2020; 8: 473.
- Hofmann A, Krufczik M, Heermann DW, Hausmann M. Using persistent homology as a new approach for super-resolution localization microscopy data analysis and classification of γH2AX foci/clusters. Int J Mol Sci 2018; 19: 2263.
- Falk M, Lukášová E, Kozubek S. Chromatin structure influences the sensitivity of DNA to gamma-radiation. Biochim Biophys Acta 2008; 1783: 2398–2414.
- Falk M, Lukášová E, Gabrielová B et al. Local changes of higher-order chromatin structure during DSB-repair. J Phys Conf Ser 2008; 101: 012018.
- Falk M, Lukášová E, Gabrielová B et al. Chromatin dynamics during DSB repair. Biochim Biophys Acta 2007; 1773: 1534–1545.
- Ježková L, Falk M, Falková I et al. Function of chromatin structure and dynamics in DNA damage, repair and misrepair: γ-rays and protons in action. Appl Radiat Isot 2014; 83 Pt B: 128–136.
- Ševčík J, Falk M, Kleiblová P et al. The BRCA1 alternative splicing variant Δ14–15 with an in-frame deletion of part of the regulatory serine-containing domain (SCD) impairs the DNA repair capacity in MCF-7 cells. Cell Signal 2012; 24: 1023–1030.
- Ševčík J, Falk M, Macurek L et al. Expression of human BRCA1Δ17–19 alternative splicing variant with a truncated BRCT domain in MCF-7 cells results in impaired assembly of DNA repair complexes and aberrant DNA damage response. Cell Signal 2013; 25: 1186–1193.
- Ježková L, Zadneprianetc M, Kulikova E et al. Particles with similar LET values generate DNA breaks of different complexity and reparability: a high-resolution microscopy analysis of γH2AX/53BP1 foci. Nanoscale 2018; 10: 1162–1179.
- Reindl J, Girst S, Walsh DWM et al. Chromatin organization revealed by nanostructure of irradiation induced γH2AX, 53BP1 and Rad51 foci. Sci Rep 2017; 7: 40616.
- Friedrich T, Ilicic K, Greubel C et al. DNA damage interactions on both nanometer and micrometer scale determine overall cellular damage. Sci Rep 2018; 8: 16063.
- Hausmann M, Wagner E, Lee JH et al. Super-resolution localization microscopy of radiation-induced histone H2AX-phosphorylation in relation to H3K9-trimethylation in HeLa cells. Nanoscale 2018; 10: 4320–4331.
- Lemmer P, Gunkel M, Baddeley D et al. SPDM: light microscopy with single-molecule resolution at the nanoscale. Appl Phys B 2008; 93: 1–12.
- Asaithamby A, Chen DJ. Mechanism of cluster DNA damage repair in response to high-atomic number and energy particles radiation. Mutat Res 2011; 711(1–2): 87–99.
- Baiocco G, Babini G, Barbieri S et al. What roles for track-structure and microdosimetry in the era of -omics and systems biology? Radiat Prot Dosimetry 2019; 183(1–2): 22–25.
- Wang H, Wang Y. Heavier ions with a different linear energy transfer spectrum kill more cells due to similar interference with the Ku-dependent DNA repair pathway. Radiat Res 2014; 182: 458–461.
Labels
Addictology Allergology and clinical immunology Angiology Audiology Clinical biochemistry Dermatology & STDs Paediatric gastroenterology Paediatric surgery Paediatric cardiology Paediatric neurology Paediatric ENT Paediatric psychiatry Paediatric rheumatology Diabetology Pharmacy Vascular surgery Pain management Dental HygienistArticle was published in
Journal of Czech Physicians
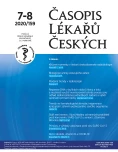
Most read in this issue
- Principles and new perspectives in the vaccination against SARS-CoV-2 virus
- Season of the year, vitamin D and COVID-19
- Age is not a disease: Evolution of protective antibodies against SARS-CoV-2 in seniors from the Břevnice nursing home
- Biological effects of ionizing radiation