Significance of aberrant DNA methylation for cancer diagnostics and therapy
Authors:
T. Urban 1,2; P. Pokorná 1,2; O. Slabý 1,2,3
Authors‘ workplace:
Biologický ústav, LF MU Brno
1; Středoevropský technologický institut, MU Brno
2; Ústav patologie, LF MU a FN Brno
3
Published in:
Klin Onkol 2024; 38(2): 88-94
Category:
Reviews
doi:
https://doi.org/10.48095/ccko202488
Overview
Background: Epigenetics is a scientific field that covers changes in gene expression that are not caused by the alteration of the nucleotide sequence in the DNA strand. Together with sequential changes, epigenetic reprogramming is a recognized cancer hallmark driving carcinogenesis. The underlying mechanisms of epigenetically-driven gene expression changes are diverse. However, one of the most extensively studied mechanisms is a change in DNA methylation. Under physiological conditions, DNA methylation ensures tissue-specific gene silencing and helps to maintain genome stability. With malignant transformation, genomic DNA undergoes global hypomethylation as well as locus-specific hypermethylation in promoters of tumor suppressor genes. In the last few decades, specific aberrant DNA methylation changes have emerged as both cancer-associated biomarkers and therapeutic targets and prompted ongoing efforts to enhance both diagnostic and therapeutic means in oncology. Purpose: The main purpose of this review is to introduce both established and emerging DNA methylation-based biomarkers for cancer diagnostics with a focus on biomarkers that are either routinely used or have been developed as commercial tests with certification for their use within in vitro diagnostics. Furthermore, therapeutic options for targeting aberrant DNA methylation are described, including both approved compounds and newly developed agents undergoing clinical investigation.
Keywords:
precision medicine – DNA methylation – Epigenetics
Sources
1. Hanahan D. Hallmarks of cancer: new dimensions. Cancer Discov 2022; 12 (1): 31–46. doi: 10.1158/2159-8290.CD-21-1059.
2. Shen H, Laird PW. Interplay between the cancer genome and epigenome. Cell 2013; 153 (1): 38–55. doi: 10.1016/j.cell.2013.03.008.
3. Lu Y, Chan Y-T, Tan H-Y et al. Epigenetic regulation in human cancer: the potential role of epi-drug in cancer therapy. Mol Cancer 2020; 19 (1): 79. doi: 10.1186/s12943-020-01197-3.
4. Das PM, Singal R. DNA methylation and cancer. J Clin Oncol 2004; 22 (22): 4632–4642. doi: 10.1200/JCO.2004.07.151.
5. Martisova A, Holcakova J, Izadi N et al. DNA methylation in solid tumors: functions and methods of detection. Int J Mol Sci 2021; 22 (8): 4247. doi: 10.3390/ijms22084247.
6. Vaisvila R, Ponnaluri VKC, Sun Z et al. Enzymatic methyl sequencing detects DNA methylation at single-base resolution from picograms of DNA. Genome Res 2021; 31 (7): 1280–1289. doi: 10.1101/gr.266551.120.
7. Herman JG, Graff JR, Myöhänen S et al. Methylation--specific PCR: a novel PCR assay for methylation status of CpG islands. Proc Natl Acad Sci U S A 1996; 93 (18): 9821–9826. doi: 10.1073/pnas.93.18.9821.
8. Wojdacz TK, Dobrovic A. Methylation-sensitive high resolution melting (MS-HRM): a new approach for sensitive and high-throughput assessment of methylation. Nucleic Acids Res 2007; 35 (6): e41. doi: 10.1093/nar/gkm013.
9. Schatz MC. Nanopore sequencing meets epigenetics. Nat Methods 2017; 14 (4): 347–348. doi: 10.1038/ nmeth.4240.
10. Clark TA, Spittle KE, Turner SW et al. Direct detection and sequencing of damaged DNA bases. Genome Integr 2011; 2: 10. doi: 10.1186/2041-9414-2-10.
11. Chen D-P, Lin Y-C, Fann CSJ. Methods for identifying differentially methylated regions for sequence- and array--based data. Brief Funct Genomics 2016; 15 (6): 485–490. doi: 10.1093/bfgp/elw018.
12. Schwarzenbach H, Hoon DSB, Pantel K. Cell-free nucleic acids as biomarkers in cancer patients. Nat Rev Cancer 2011; 11 (6): 426–437. doi: 10.1038/nrc3066.
13. Usadel H, Brabender J, Danenberg KD et al. Quantitative adenomatous polyposis coli promoter methylation analysis in tumor tissue, serum, and plasma DNA of patients with lung cancer. Cancer Res 2002; 62 (2): 371–375.
14. Bearzatto A, Conte D, Frattini M et al. p16 (INK4A) Hypermethylation detected by fluorescent methylation-specific PCR in plasmas from non-small cell lung cancer. Clin Cancer Res 2002; 8 (12): 3782–3787.
15. Weiss G, Schlegel A, Kottwitz D et al. Validation of the SHOX2/PTGER4 DNA methylation marker panel for plasma-based discrimination between patients with malignant and nonmalignant lung disease. J Thorac Oncol 2017; 12 (1): 77–84. doi: 10.1016/j.jtho.2016.08.123.
16. Lin KW. mSEPT9 (Epi proColon) blood test for colorectal cancer screening. Am Fam Physician 2019; 100 (1): 10–11.
17. Imperiale TF, Ransohoff DF, Itzkowitz SH et al. Multitarget stool DNA testing for colorectal-cancer screening. N Engl J Med 2014; 370 (14): 1287–1297. doi: 10.1056/NEJMoa1311194.
18. FDA. List of cleared or approved companion diagnostic devices (in vitro and imaging tools). [online]. Dostupné z: https: //www.fda.gov/medical-devices/in-vitro-diagnostics/list-cleared-or-approved-companion-diagnostic-devices-in-vitro-and-imaging-tools.
19. Barták BK, Kalmár A, Péterfia B et al. Colorectal adenoma and cancer detection based on altered methylation pattern of SFRP1, SFRP2, SDC2, and PRIMA1 in plasma samples. Epigenetics 2017; 12 (9): 751–763. doi: 10.1080/15592294.2017.1356957.
20. Clarke CA, Hubbell E, Ofman JJ. Multi-cancer early detection: a new paradigm for reducing cancer-specific and all-cause mortality. Cancer Cell 2021; 39 (4): 447–448. doi: 10.1016/j.ccell.2021.02.004.
21. Klein EA, Richards D, Cohn A et al. Clinical validation of a targeted methylation-based multi-cancer early detection test using an independent validation set. Ann Oncol 2021; 32 (9): 1167–1177. doi: 10.1016/j.annonc.2021.05.806.
22. Witjes JA, Morote J, Cornel EB et al. Performance of the bladder EpiCheckTM methylation test for patients under surveillance for non-muscle-invasive bladder cancer: results of a multicenter, prospective, blinded clinical trial. Eur Urol Oncol 2018; 1 (4): 307–313. doi: 10.1016/j.euo.2018.06.011.
23. Lin H, Chen T-C, Chang T-C et al. Methylated ZNF582 gene as a marker for triage of women with Pap smear reporting low-grade squamous intraepithelial lesions – a Taiwanese Gynecologic Oncology Group (TGOG) study. Gynecol Oncol 2014; 135 (1): 64–68. doi: 10.1016/j.ygyno.2014.08.012.
24. Kan Y-Y, Liou Y-L, Wang H-J et al. PAX1 methylation as a potential biomarker for cervical cancer screening. Int J Gynecol Cancer 2014; 24 (5): 928–934. doi: 10.1097/IGC.0000000000000155.
25. Schmitz M, Eichelkraut K, Schmidt D et al. Performance of a DNA methylation marker panel using liquid--based cervical scrapes to detect cervical cancer and its precancerous stages. BMC Cancer 2018; 18 (1): 1197. doi: 10.1186/s12885-018-5125-8.
26. Moran S, Martínez-Cardús A, Sayols S et al. Epigenetic profiling to classify cancer of unknown primary: a multicentre, retrospective analysis. Lancet Oncol 2016; 17 (10): 1386–1395. doi: 10.1016/S1470-2045 (16) 30 \\\297-2.
27. Capper D, Jones DTW, Sill M et al. DNA methylation-based classification of central nervous system tumours. Nature 2018; 555 (7697): 469–474. doi: 10.1038/nature26000.
28. Koelsche C, Schrimpf D, Stichel D et al. Sarcoma classification by DNA methylation profiling. Nat Commun 2021; 12 (1): 498. doi: 10.1038/s41467-020-20603-4.
29. Louis DN, Perry A, Wesseling P et al. The 2021 WHO classification of tumors of the central nervous system: a summary. Neuro Oncol 2021; 23 (8): 1231–1251. doi: 10.1093/neuonc/noab106.
30. Jaunmuktane Z, Capper D, Jones DTW et al. Methylation array profiling of adult brain tumours: diagnostic outcomes in a large, single centre. Acta Neuropathol Commun 2019; 7 (1): 24. doi: 10.1186/s40478-019-0668-8.
31. Kuschel LP, Hench J, Frank S et al. Robust methylation-based classification of brain tumours using nanopore sequencing. Neuropathol Appl Neurobiol 2023; 49 (1): e12856. doi: 10.1111/nan.12856.
32. Vermeulen C, Pagès-Gallego M, Kester L et al. Ultra-fast deep-learned CNS tumour classification during surgery. Nature 2023; 622 (7984): 842–849. doi: 10.1038/s41586-023-06615-2.
33. Quillien V, Lavenu A, Ducray F et al. Clinical validation of the CE-IVD marked Therascreen MGMT kit in a cohort of glioblastoma patients. Cancer Biomark 2017; 20 (4): 435–441. doi: 10.3233/CBM-170191.
34. Weller M, Felsberg J, Hartmann C et al. Molecular predictors of progression-free and overall survival in patients with newly diagnosed glioblastoma: a prospective translational study of the German glioma network. J Clin Oncol 2009; 27 (34): 5743–5750. doi: 10.1200/JCO.2009.23.0805.
35. Wick W, Platten M, Meisner C et al. Temozolomide chemotherapy alone versus radiotherapy alone for malignant astrocytoma in the elderly: the NOA-08 randomised, phase 3 trial. Lancet Oncol 2012; 13 (7): 707–715. doi: 10.1016/S1470-2045 (12) 70164-X.
36. Malmström A, Grønberg BH, Marosi C et al. Temozolomide versus standard 6-week radiotherapy versus hypofractionated radiotherapy in patients older than 60 years with glioblastoma: the Nordic randomised, phase 3 trial. Lancet Oncol 2012; 13 (9): 916–926. doi: 10.1016/S1470-2045 (12) 70265-6.
37. Mansouri A, Hachem LD, Mansouri S et al. MGMT promoter methylation status testing to guide therapy for glioblastoma: refining the approach based on emerging evidence and current challenges. Neuro Oncol 2019; 21 (2): 167–178. doi: 10.1093/neuonc/noy132.
38. Schmitt M, Wilhelm OG, Noske A et al. Clinical validation of PITX2 DNA methylation to predict outcome in high-risk breast cancer patients treated with anthracycline-based chemotherapy. Breast Care (Basel) 2018; 13 (6): 425–433. doi: 10.1159/000493016.
39. Scott LJ. Azacitidine: a review in myelodysplastic syndromes and acute myeloid leukaemia. Drugs 2016; 76 (8): 889–900. doi: 10.1007/s40265-016-0585-0.
40. Jones PA, Taylor SM. Cellular differentiation, cytidine analogs and DNA methylation. Cell 1980; 20 (1): 85–93. doi: 10.1016/0092-8674 (80) 90237-8.
41. Garcia JS, Jain N, Godley LA. An update on the safety and efficacy of decitabine in the treatment of myelodysplastic syndromes. Onco Targets Ther 2010; 3: 1–13. doi: 10.2147/ott.s7222.
42. Gu X, Tohme R, Tomlinson B et al. Decitabine- and 5-azacytidine resistance emerges from adaptive responses of the pyrimidine metabolism network. Leukemia 2021; 35 (4): 1023–1036. doi: 10.1038/s41375-020-1003-x.
43. Stomper J, Rotondo JC, Greve G et al. Hypomethylating agents (HMA) for the treatment of acute myeloid leukemia and myelodysplastic syndromes: mechanisms of resistance and novel HMA-based therapies. Leukemia 2021; 35 (7): 1873–1889. doi: 10.1038/s41375-021- 01218-0.
44. Romero-Garcia S, Prado-Garcia H, Carlos-Reyes A. Role of DNA methylation in the resistance to therapy in solid tumors. Front Oncol 2020; 10: 1152. doi: 10.3389/fonc.2020.01152.
45. Dai M, Liu M, Yang H et al. New insights into epigenetic regulation of resistance to PD-1/PD-L1 blockade cancer immunotherapy: mechanisms and therapeutic opportunities. Exp Hematol Oncol 2022; 11 (1): 101. doi: 10.1186/s40164-022-00356-0.
46. Daher-Reyes GS, Merchan BM, Yee KWL. Guadecitabine (SGI-110): an investigational drug for the treatment of myelodysplastic syndrome and acute myeloid leukemia. Expert Opin Investig Drugs 2019; 28 (10): 835–849. doi: 10.1080/13543784.2019.1667331.
47. Roboz GJ, Sanz G, Griffiths EA et al. Results from a global randomized phase 3 study of guadecitabine (G) vs treatment choice (TC) in 302 patients with relapsed or refractory (r/r) acute myeloid leukemia after intensive chemotherapy (ASTRAL-2 study). Blood 2021; 138 (Suppl 1): 2344. doi: 10.1182/blood-2021-147769.
48. O’Sullivan Coyne G, Wang L, Zlott J et al. Intravenous 5-fluoro-2’-deoxycytidine administered with tetrahydrouridine increases the proportion of p16-expressing circulating tumor cells in patients with advanced solid tumors. Cancer Chemother Pharmacol 2020; 85 (5): 979–993. doi: 10.1007/s00280-020-04073-5.
49. Nguyen J, O’Sullivan Coyne G, Takebe N et al. Phase I trial of 5-aza-4’-thio-2’-deoxycytidine (Aza-TdC) in patients with advanced solid tumors. J Clin Oncol 2021; 39 (Suppl 15): 3088. doi: 10.1200/JCO.2021.39.15_suppl.3088.
50. Arce C, Segura-Pacheco B, Perez-Cardenas E et al. Hydralazine target: from blood vessels to the epigenome. J Transl Med 2006; 4: 10. doi: 10.1186/1479-5876-4-10.
51. Fang MZ, Wang Y, Ai N et al. Tea polyphenol (-) -epigallocatechin-3-gallate inhibits DNA methyltransferase and reactivates methylation-silenced genes in cancer cell lines. Cancer Res 2003; 63 (22): 7563–7570.
52. Huang S, Stillson NJ, Sandoval JE et al. A novel class of selective non-nucleoside inhibitors of human DNA methyltransferase 3A. Bioorg Med Chem Lett 2021; 40: 127908. doi: 10.1016/j.bmcl.2021.127908.
53. Davis AJ, Gelmon KA, Siu LL et al. Phase I and pharmacologic study of the human DNA methyltransferase antisense oligodeoxynucleotide MG98 given as a 21-day continuous infusion every 4 weeks. Invest New Drugs 2003; 21 (1): 85–97. doi: 10.1023/a: 1022976528441.
Labels
Paediatric clinical oncology Surgery Clinical oncologyArticle was published in
Clinical Oncology
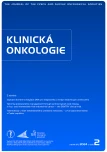
2024 Issue 2
Most read in this issue
- Significance of aberrant DNA methylation for cancer diagnostics and therapy
- Carcinoid syndrome with right-sided valve involvement – a case report and review of the literature
- Feasibility of implementation of the early tumor shrinkage as a potential predictive marker to daily clinical practice in patients with RAS wild type metastatic colorectal cancer, treated with cetuximab – a non-interventional observational study
- Factors influencing overall survival and GvHD development after allogeneic hematopoietic stem cell transplantation – single centre experience