Synthetic Lethality – Its Current Application and Potential in Oncological Treatment
Authors:
L. Bortlíková 1; P. Müller 1; B. Vojtěšek 1; V. Rak 2; M. Svoboda 3
Authors‘ workplace:
Regionální centrum aplikované molekulární onkologie, Masarykův onkologický ústav, Brno
1; Klinika radiační onkologie, Masarykův onkologický ústav, Brno
2; Klinika komplexní onkologické péče, Masarykův onkologický ústav, Brno
3
Published in:
Klin Onkol 2019; 32(Supplementum 3): 19-24
Category:
Review
doi:
https://doi.org/10.14735/amko20193S
Overview
Background: Synthetic lethality is a gene interaction where a defect in one of the interacting genes is compatible with cell viability, whereas the disruption of both genes leads to cell death. The discovery of the lethal effect of poly(ADP-ribose) polymerase (PARP) inhibitors in BRCA1/2 mutant cells has opened an important direction in the development of targeted therapy in oncology. The PARP inhibitor olaparib has become the first registered drug for recurrent high-grade serous ovarian cancer treatment based on synthetic lethality that has reached the clinic. Current research focuses on the combination of PARP inhibitors and inhibitors of kinases, which control the cell cycle, to prevent or overcome resistance to PARP inhibitors. There are also ongoing clinical trials which examine PARP inhibitor treatment in other types of cancers including tumours presenting the so-called BRCAness phenotype. Screenings for new synthetic lethalities which could serve as potential targets for new drug development have improved with the CRISPR/Cas9 technology, but another key problem persists in the screening efforts, namely the incomplete penetrance of synthetic lethality throughout a tumour cell population.
Purpose: This paper summarises the current application of synthetic lethality principles in oncology and discusses the challenges in research focused on potential new drugs based on synthetic lethality.
Keywords:
synthetic lethality – PARP inhibitors – olaparib – BRCAness – DNA repair
Sources
1. Ashworth A, Lord CJ, Reis-Filho J. Genetic interactions in cancer progression and treatment. Cell 2011; 145(1): 30–38. doi: 10.1016/ j.cell.2011.03.020.
2. Hartwell LH, Szankasi P, Roberts CJ et al. Integrating genetic approaches into the discovery of anticancer drugs. Science 1997; 278(5340): 1064–1068. doi: 10.1126/ science.278.5340.1064.
3. Kaelin WG Jr. The concept of synthetic lethality in the context of anticancer therapy. Nat Rev Cancer 2005; 5(9): 689–698. doi: 10.1038/ nrc1691.
4. Brummelkamp TR, Bernards R. New tools for functional mammalian cancer genetics. Nat Rev Cancer 2003; 3(10): 781–789. doi: 10.1038/ nrc1191.
5. Ashworth A, Lord CJ. Synthetic lethal therapies for cancer: what’s next after PARP inhibitors? Nat Rev Clin Oncol 2018; 15(9): 564–576. doi: 10.1038/ s41571-018-0055-6.
6. Farmer H, McCabe N, Lord CJ et al. Targeting the DNA repair defect in BRCA mutant cells as a therapeutic strategy. Nature 2005; 434(7035): 917–921. doi: 10.1038/ nature03445.
7. Bryant HE, Schultz N, Thomas HD et al. Specific killing of BRCA2-deficient tumours with inhibitors of poly(ADP-ribose) polymerase. Nature 2005; 434(7035): 913–917. doi: 10.1038/ nature03443.
8. Lord CJ, Ashworth A. PARP inhibitors: Synthetic lethality in the clinic. Science 2017; 355(6330): 1152–1158. doi: 10.1126/ science.aam7344.
9. Minchom A, Aversa C, Lopez J. Dancing with the DNA damage response: next-generation anti-cancer therapeutic strategies. Ther Adv Med Oncol 2018; 10: 1–18. doi: 10.1177/ 1758835918786658.
10. Audeh MW, Carmichael J, Penson RT et al. Oral poly(ADP-ribose) polymerase inhibitor olaparib in patients with BRCA1 or BRCA2 mutations and recurrent ovarian cancer: a proof-of-concept trial. Lancet 2010; 376(9737): 245–251. doi: 10.1016/ S0140-6736(10)60893-8.
11. Gelmon KA, Tischkowitz M, Mackay H et al. Olaparib in patients with recurrent high-grade serous or poorly differentiated ovarian carcinoma or triple-negative breast cancer: a phase 2, multicentre, open-label, non-randomised study. Lancet Oncol 2011; 12(9): 852–861. doi: 10.1016/ S1470-2045(11)70214-5.
12. Evans T, Matulonis U. PARP inhibitors in ovarian cancer: evidence, experience and clinical potential. Ther Adv Med Oncol 2017; 9(4): 253–267. doi: 10.1177/ 1758834016687254.
13. Turk AA, Wisinski KB. PARP inhibitors in breast cancer: Bringing synthetic lethality to the bedside. Cancer 2018; 124(12): 2498–2506. doi: 10.1002/ cncr.31307.
14. Sakai W, Swisher EM, Karlan BY et al. Secondary mutations as a mechanism of cisplatin resistance in BRCA2-mutated cancers. Nature 2008; 451(7182): 1116–1120. doi: 10.1038/ nature06633.
15. Jaspers JE, Kersbergen A, Boon U et al. Loss of 53BP1 causes PARP inhibitor resistance in BRCA1-mutated mouse mammary tumors. Cancer Discov 2013; 3(1): 68–81. doi: 10.1158/ 2159-8290.CD-12-0049.
16. Xu G, Chapman JR, Brandsma I et al. REV7 counteracts DNA double-strand break resection and affects PARP inhibition. Nature 2015; 521(7553): 541–544. doi: 10.1038/ nature14328.
17. Ghezraoui H, Oliveira C, Becker JR et al. 53BP1 cooperation with the REV7-shieldin complex underpins DNA structure-specific NHEJ. Nature 2018; 560(7716):122–127. doi: 10.1038/ s41586-018-0362-1.
18. Noordermeer SM, Adam S, Setiaputra D et al. The shieldin complex mediates 53BP1-dependent DNA repair. Nature 2018; 560(7716): 117–121. doi: 10.1038/ s41586-018-0340-7.
19. Dev H, Chiang TW, Lescale C et al. Shieldin complex promotes DNA end-joining and counters homologous recombination in BRCA1-null cells. Nat Cell Biol 2018; 20(8): 954–965. doi: 10.1038/ s41556-018-0140-1.
20. Liu X, Han EK, Anderson M et al. Acquired resistance to combination treatment with temozolomide and ABT-888 is mediated by both base excision repair and homologous recombination DNA repair pathways. Mol Cancer Res 2009; 7(10): 1686–1692. doi: 10.1158/ 1541-7786.MCR-09-0299.
21. Volinia S, Galasso M, Sana ME et al. Breast cancer signatures for invasiveness and prognosis defined by deep sequencing of microRNA. Proc Natl Acad Sci USA 2012; 109(8): 3024–3029. doi: 10.1073/ pnas.1200010109.
22. Du Y, Yamaguchi H, Hsu JL et al. PARP inhibitors as precision medicine for cancer treatment. Natl Sci Rev 2017; 4(4): 576–592. doi: 10.1093/ nsr/ nwx027.
23. Gilabert M, Launay S, Ginestier C et al. Poly(ADP-ribose) polymerase 1 (PARP1) overexpression in human breast cancer stem cells and resistance to olaparib. PLoS One 2014; 9(8): e104302. doi: 10.1371/ journal.pone.0104302.
24. Durmus S, Sparidans RW, van Esch A et al. Breast cancer resistance protein (BCRP/ ABCG2) and P-glycoprotein (P-GP/ ABCB1) restrict oral availability and brain accumulation of the PARP inhibitor rucaparib (AG-014699). Pharm Res 2015; 32(1): 37–46. doi: 10.1007/ s11095-014-1442-z.
25. Vaidyanathan A, Sawers L, Gannon AL et al. ABCB1 (MDR1) induction defines a common resistance mechanism in paclitaxel- and olaparib-resistant ovarian cancer cells. Br J Cancer 2016; 115(4): 431–441. doi: 10.1038/ bjc.2016.203.
26. Maya-Mendoza A, Moudry P, Merchut-Maya JM et al. High speed of fork progression induces DNA replication stress and genomic instability. Nature 2018; 559(7713): 279–284. doi: 10.1038/ s41586-018-0261-5.
27. Schlacher K, Wu H, Jasin, M. A distinct replication fork protection pathway connects Fanconi anemia tumor suppressors to RAD51-BRCA1/ 2. Cancer Cell 2012; 22(1): 106–116. doi: 10.1016/ j.ccr.2012.05.015.
28. Haynes B, Murai J, Lee JM. Restored replication fork stabilization, a mechanism of PARP inhibitor resistance, can be overcome by cell cycle checkpoint inhibition. Cancer Treat Rev 2018; 71: 1–7. doi: 10.1016/ j.ctrv.2018.09.003.
29. Yekezare M, Gómez-González B, Diffley JF. Controlling DNA replication origins in response to DNA damage - inhibit globally, activate locally. J Cell Sci 2013; 126(6): 1297–1306. doi: 10.1242/ jcs.096701.
30. Mechali M. Eukaryotic DNA replication origins: many choices for appropriate answers. Nat Rev Mol Cell Biol 2010; 11(10): 728–738. doi: 10.1038/ nrm2976.
31. Carrassa L, Damia G. DNA damage response inhibitors: mechanisms and potential applications in cancer therapy. Cancer Treat Rev 2017; 60: 139–151. doi: 10.1016/ j.ctrv.2017.08.013.
32. Sanjiv K, Hagenkort A, Calderon-Montano JM et al. Cancer-specific synthetic lethality between ATR and CHK1 kinase activities. Cell Reports 2016; 17(12): 3407–3416. doi: 10.1016/ j.celrep.2016.12.031.
33. Magnussen GI, Emilsen E, Giller Fleten K et al. Combined inhibition of the cell cycle related proteins Wee1 and Chk1/ 2 induces synergistic anti-cancer effect in melanoma. BMC Cancer 2015; 15: 462. doi: 10.1186/ s12885-015-1474-8.
34. Guertin AD, Martin MM, Roberts B et al. Unique functions of CHK1 and WEE1 underlie synergistic anti-tumor activity upon pharmacologic inhibition. Cancer Cell Int 2012; 12(1): 45. doi: 10.1186/ 1475-2867-12-45.
35. Toledo LI, Altmeyer M, Rask MB et al. ATR prohibits replication catastrophe by preventing global exhaustion of RPA. Cell 2013; 155(5): 1088–1103. doi: 10.1016/ j.cell.2013.10.043.
36. Elvers I, Hagenkort A, Johansson F et al. CHK1 activity is required for continuous replication fork elongation but not stabilization of post-replicative gaps after UV irradiation. Nucleic Acids Res 2012; 40(17): 8440–8448. doi: 10.1093/ nar/ gks646.
37. Couch FB, Bansbach CE, Driscoll R et al. ATR phosphorylates SMARCAL1 to prevent replication fork collapse. Genes Dev 2013; 27(14): 1610–1623. doi: 10.1101/ gad.214080.113.
38. Kim H, George E, Ragland R et al. Targeting the ATR/ CHK1 Axis with PARP inhibition results in tumor regression in BRCA-mutant ovarian cancer models. Clin Cancer Res 2017; 23(12): 3097–3108. doi: 10.1158/ 1078-0432.CCR-16-2273.
39. Dominguez-Kelly R, Martin Y, Koundrioukoff S et al. Wee1 controls genomic stability during replication by regulating the Mus81-Eme1 endonuclease. J Cell Biol 2011; 194(4): 567–579. doi: 10.1083/ jcb.201101047.
40. Lord CJ, Ashworth A. BRCAness revisited. Nat Rev Cancer 2016; 16(2): 110–120. doi: 10.1038/ nrc.2015.21.
41. Waddell N, Pajic M, Patch AM et al. Whole genomes redefine the mutational landscape of pancreatic cancer. Nature 2015; 518(7540): 495–501. doi: 10.1038/ nature14169.
42. Robinson D, Van Allen EM, Wu YM et al. Integrative clinical genomics of advanced prostate cancer. Cell 2015; 161(5): 1215–1228. doi: 10.1016/ j.cell.2015.05.001.
43. Severson TM, Wolf DM, Yau C et al. The BRCA1ness signature is associated significantly with response to PARP inhibitor treatment versus control in the I-SPY 2 randomized neoadjuvant setting. Breast Cancer Res 2017; 19(1): 99. doi: 10.1186/ s13058-017-0861-2.
44. Ryan CJ, Bajrami I, Lord CJ. Synthetic lethality and cancer – penetrance as the major barrier. Trends Cancer 2018; 4(10): 671–683. doi: 10.1016/ j.trecan.2018.08.003.
45. Martin TD, Cook DR, Choi MY et al. A role for mitochondrial translation in promotion of viability in K-Ras mutant cells. Cell Rep 2017; 20(2): 427–438. doi: 10.1016/ j.celrep.2017.06.061.
46. Shen JP, Zhao D, Sasik R et al. Combinatorial CRISPR–Cas9 screens for de novo mapping of genetic interactions. Nat Methods 2017; 14(6): 573–576. doi: 10.1038/ nmeth.4225.
47. Wang T, Yu H, Hughes NW et al. Gene essentiality profiling reveals gene networks and synthetic lethal interactions with oncogenic Ras. Cell 2017; 168(5): 890–903. doi: 10.1016/ j.cell.2017.01.013.
Labels
Paediatric clinical oncology Surgery Clinical oncologyArticle was published in
Clinical Oncology
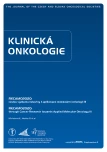
2019 Issue Supplementum 3
Most read in this issue
- Protein Ubiquitination Research in Oncology
- CRISPR-Cas9 as a Tool in Cancer Therapy
- Glycosylation as an Important Regulator of Antibody Function
- Uncommon EGFR Mutations in Non-Small Cell Lung Cancer and Their Impact on the Treatment