Monitoring of EMG to force ratio using new designed precise wireless sensor system
Authors:
Erik Vavrinsky 1; Martin Donoval 1,2; Martin Daricek 1; Frantisek Horinek 1; Marian Popovic 1; Michal Hanic 1; Martin Jagelka 1
Authors‘ workplace:
Institute of Electronics and Photonics, Slovak University of Technology, Ilkovicova 3, 81 19 Bratislava, Slovakia
1; NanoDesign ltd., Drotarska 19A, 811 04 Bratislava, Slovakia
2
Published in:
Lékař a technika - Clinician and Technology No. 3, 2014, 44, 17-22
Category:
Original research
Overview
This work is focused on problematic of biopotential signals measurements (EXG) using powerful SMART sensor system, composed of portable units, intended for in-time wireless measurement and evaluation of electrical activity, produced by skeletal muscles, human heart or brain. Here, we discuss very precise measurement features, which characterize this device (high gain, low noise, wireless data transfer, multi-probe measuring), some special features as low voltage and ultra-low power consumption were reached by application of the described amplifier in order to achieve its longer performance for daily use. It brings a lot of advantages to biomedical electronics and medical care. In order to optimize the performance of novel proposed smart biomedical instrument in our experimental part we have focused on measurement of surface electromyography (sEMG) signal to force ratio. These sEMG signals can illuminate our understanding of how the brain controls muscles to generate force and produce movement and can be used in such applications like as training of athletes, controlling robots, monitoring the physical capabilities of patients with motor disorders etc.
Keywords:
surface electromyography, EMG to force ratio, 2 – electrode configuration, Wireless sensor
Introduction
Interconnection of microelectronics and medicine has been particularly in last year’s very interesting technical field. Increasing of computing power of new microprocessors, production of novel integrated chips and continual development of measurable human health parameters sensing technics positively impacts the expansion of new integrated medical devices. These health monitoring devices present powerful healthcare assistance for end consumer, but also useful instrument for many biomedical research groups.
This paper reviews designs of instrumentation used in biopotential measurements, suitable in particular for the electromyogram (EMG), the electrocardiogram (ECG) and the electroencephalogram (EEG) signals. The biopotentials are acquired with specialized electrodes that interface to the organ or the body and transduce low-noise, artefact-free signals. The basic design of a biopotential amplifier consists of an instrumentation amplifier. The amplifier should pos-sess several characteristics, including high amplifica-tion, input impedance, and the ability to reject electrical interference, all of which are needed for the measurement of these biopotentials. Ancillary circuits are used in order to attenuating or prevent electric interference, electrical isolation, and defibrillation shock [1]. In order to characterize the performance power of novel proposed smart biomedical instrument in the experiment part we have focused on measurement of surface electromyography (sEMG) to human force ratio.
Surface electromyography (sEMG) is a measurement method of the electrical potential, present on the skin as a consequence of muscle contraction. This voltage is detected by electrodes placed on the skin. Upon specific signal filtering and a use of proper protocol for electrode placement is the voltage, measured on the skin, related to the activity of a single specific muscle. The signal is represented as a trace relative on time, increasing from zero up to tenths or hundreds of microvolts as the muscle become activated. sEMG can be described as the study of the neuromuscular activation of muscles within postural tasks, functional movements, work conditions and treatment/training regimes. It allows the investigation of both muscle activation and muscle physiological characteristics. Besides basic physiological and biomechanical studies a sEMG can be used as an evaluation tool for applied research, physiotherapy/rehabilitation, in motion analysis to assess superficial muscle function, sports training and interactions of the human body to industrial products and work conditions [2, 3].
EMG activation is the preliminary condition for any force development and very close relationship between both measures can be expected. Indeed, there is a very high correlation between both parameters; unfortunately it can greatly vary within its characteristics. For the estimation of muscles force there is not a simple and reliable method mostly because usually several muscles take part in a single motion. For direct measurement of muscle forces, electromyography (EMG) method has been frequently applied as a standard clinical tool in identification of activation level of muscles, but in this method the muscle activity result often results in ratio of percents rather than quantitative values in Newton units [4, 5]. Moreover, the surface EMG methods are suitable for measurement of surface muscles activity only. The typical case is a curve-linear relationship: at higher force proportionally higher EMG ration is related to force increase. For certain conditions within static force testing some (smaller) muscles tend to show true linear EMG-Force relationship. The investigation of such relationships is important if the EMG signal is used in torque calculations within biomechanical models. Under a more practical point of view, e.g. within clinical treatment procedures, one can safely derive that with any EMG increase the torque and compression force around a joint increases in a similar manner. The EMG-Force ratio can be used to determine the neuromuscular (training) status of a muscle [3]. Measured EMG signals are often used as control signals for prosthetic devices such as prosthetic hands, arms, and lower limbs. With new low-noise technologies these method can be used to sense even isometric muscular activity at no muscle movement, which significant impacts to the treatment process of neural and neuromuscular diseases. The detected signals can be used as well for other practical purposes, as to control electronic devices such as assisting robotic hands, mobile phones or PDA [6 - 9].
The use of EMG signal as a gauge for determining the force is both fascinating and challenging. It is fascinating because of the possibilities for making a quantitative measure of strength in an individual while performing a motion, through non-invasive surface electrodes. It is challenging in view of the complexity and variability inherent in biological signals, especially in dynamic situations. Environmental electric interference is always present, especially in urban hospital environments. It is desirable to eliminate interference before it enters the amplifier, for example, by proper shielding and grounding of the subject and the instrument. Sources of interference include induced signals from power lines and electric wiring; RF from transmitters, electric motors, and other appliances; magnetically induced currents in lead wires; and others [10].
Methods
Proposed sensor system is composed of autonomous sensing units, dedicated for wireless real-time bioelectrical potential measurement and evaluation (Fig. 1), with simultaneous monitoring of different body parts at the same time.
The sensing unit is based on precise instrumentation amplifier designed for bipolar detection arrangement. The amplified bio-signals are sampled by 16 bit analogue-digital converter (ADC), pre-processed by microcontroller and sent via RF-bridge to computer for further digital processing (DSP). Dedicated input amplifier for EXG measurement is characterized by low supply voltage, high common mode rejection (CMR) and rail-to-rail output. The designed concept solves many of the typical challenges of EXG signals measurements. Potentials measured on the skin may vary in scale of nanovolts to millivolts. The measured voltage depends on several parameters, for example the type of the muscle and the position of the sensors. For this reason a variable gain (60 or 80 dB) was implemented in the amplifier solution. The sensing unit was intended to be at high versatility level. Most of EMG and ECG experiments require gain of 60 dB; however at EEG measuring it is necessary to employ a gain of 80 dB. Common-mode signal, transferred from ambient electromagnetic energy and electrical lines helps to reject high CMR by 100dB. The human body behaves like a big antenna receiving ambient electromagnetic signals, particularly at frequency of 50 Hz, induced by ambient main power supply. These signals are in the EMG frequency range and should be suppressed to minimum. The rail-to-rail output, provided by 20 dB gain, offers sufficient dynamic range at battery power supply. Output of the instrumentation amplifier is equipped by an active band pass filter, actually with pass band of 1 Hz – 1 kHz. The high pass part of the filter works as the feedback to body reference electrode and effectively suppress ineligible frequencies. Corner frequency of low pass part is set to 1 kHz [11–13]. After the analogue processing stage is [14] the signal is prepared for precise 16 bit AD conversion at rate of 13.33 kHz (maximum of 100 kHz at high-SR mode, reset by receiving unit) sample rate. Digitalized data are further transferred to microcontroller with integrated RF communication block, where they are pre-processed and sent to main CPU of the system via RF-bridge for essential DSP processing. The system further buffers, inverts and amplifies the common voltage, taken at the midpoint of the gain setting resistor. Common-mode signals are rejected by active reference drive circuit integrated to body reference electrode. Power consumption of designed prototype of RF sensor system is less than 10 mA. The whole portable RF amplifier is battery powered by Li-Pol accumulator.
In [7] is described that for DSP of EMG signals it is ideal to use minimal filtering and to measure in full signal spectrum. According to our research there is a need of strong mathematical post-processing (for each muscle block individual band-pass filter setting and calibrating) for any practical purpose (e.g. processing of bio-signals for controlling of prosthetic devices). Generally, we have observed that surface EMG filter frequency settings are dependent on size of muscles group. For small muscles it is better to use low frequency filters and for greater muscle groups, higher frequencies are required (e.g. flexor carpum: 55–95 Hz, biceps femoris and pectoralis major: 155–195 Hz).
Used electrodes were constructed in double-sided PCB technology on FR4 substrate, with passive gold plated surface. All the electrodes are designed in 2 electrode configuration. The advantageous solution brings more scientific options and allows further electrode miniaturization. In described experiments we used strip geometry electrodes with 3 different sizes.
Dedicated wireless portable biomedical amplifier helped to detach the sensors of the lead wires and to remove the noise introduced by pre-amplifier stage. The results also confirmed that at small dimension electrodes bio-measurement there is no need for use of conductive gel [15]. Previously obtained results clearly proved very high quality, stability and well noise cancellation (approximately 5-time lower noise level) of dedicated wireless sensing system with better measurement results in comparison to other conventionally used devices. We also gained much better minimization of unfavourable input artefacts. Our system is easier to use with cordless setup without application of conductive gels and other advance preparations methods, based on principle place and measure.
Results
Measuring of EMG to force ratio
The relationship between EMG and human force was measured by strip electrodes (Fig. 1d) and EXG sensor system placed on gastroc-nemius (Fig. 2). The gas-trocnemius practice, heel raise, is very suitable for calculation of EMG to force ratio, because we achieved very constant load at each heel raise in time with the force vector at relatively constant level (isometric measurement). The electro-des were placed on human body continuously during whole experiment.
Proband practice consis-ted always of series of 5 heel raises with constant load, followed by proper relaxation time (to minimize fatigue factor), next series of 5 heel raises with increased load, and so on. We started with very small load about 1000 N (proband weight apx.90 kg included) and ended with load 1850 N. Each electrode configuration was tested on different day to avoid fatigue influence. Measured EMG signal for B1 electrode configuration in bandwidth 155–195 Hz is to see in Fig. 3. EMG values of all electrode configurations in Fig. 5a were obtained from averaged peak maxims. This became possible because we strongly controlled proband practice speed, so the heel raise time was constant. For isometric EMG to force ratio - EMG=f(F) we obtained linear relationships that corresponds to [16-18]:
electrode B1: EMG = 45 * 10-9 * F,
electrode B2: EMG = 58 * 10-9 * F,
electrode B3: EMG = 91 * 10-9 * F,
where EMG is amplitude in V and F is corresponded force in N.
Considering the automated signal evaluation aim and development of control algorithms for specialized actuators, like prosthetic devices and robots is more suitable parameter the “Total power” of EMG spectrum [3].
The Total power spectrum (Fig. 4) was calculated in program Labchart [19] using following parameters: FFT size = 8k, which corresponds to 0,6 second actuator sampling speed, Data window = Hann (cosine-bell), Window overlap = 93,75%. For Total power spectrum of EMG (Fig. 5b) on force dependence we obtained expected quadratic relationships:
electrode B1: Total Power = 293 * 10-6 * F2,
electrode B2: Total Power = 428 * 10-6 * F2,
electrode B3: Total Power = 945 * 10-6 * F2
where Total power is amplitude of Total power of EMG spectrum in μV2 and F is corresponded force N. This function was fully automatically calculated and is ideal for processing of bio-signals for controlling of prosthetic devices.
As you see the direction constants in relationship functions of EMG and Total power spectrum of EMG are quasi linear dependent on electrode notch. The aberrances are caused local body parameter changes (skin resistance, blood vessels impedance and sweat capillaries properties) and in order to achieve higher accuracy an addition of “rectangular to planar” factor into the calculations is required. This relationship will be investigated in detail in later researches.
Fatigue influence
Fatigue influence to our force ratio measurement was investigated on periodical heel raises in time of 80 seconds with constant load F = 1619 N. EMGMEAN was automatically calculated in Labchart [19] from absolute value of filtered EMG in bandwidth 155–195 Hz, where the threshold value was set to EMG = 15μV. The value was averaged from EMG signal between two contractions. Obtained fatigue as linear time function is:
EMGMEAN= 5,96 + 0,03 * Time,
which implicates that each heel raise series on near to maximum load without relaxation phase increases amplitude of EMG for about 5 %. Therefore by including relaxation phases between each series and starting with much lower load is the influence of fatigue to our previous experiments results under 1% and can be ignored.
Conclusion
High quality and scientific potential of the designed smart EXG sensor system was experimentally proven. Proposed smart system achieves user friendly features with cordless sensing units with no need of application of conductive gels and other advanced preparations methods. Together with ultra-low power consumption the novel device provides a very suitable and promising long-term testing and medical surveillance means for development of new healthcare diagnostic methods.
We have demonstrated the functionality of our system by measurement of the relationship between electrical activity and isometric muscle force, which can be applied in kinesiology or to control electrical devices such as Smart-phones, machines or prosthetic body-parts.
The electrical conductivity despite a good conductivity of human body unfortunately varies with tissue type, thickness, physiological changes and temperature. These conditions can greatly vary from subject to subject (and even within subject) and prohibit a direct quantitative comparison of EMG amplitude parameters calculated on the unprocessed EMG signal. Therefore the system modularity and open measurement parameters setup presents another advantage of the described system, which creates a large potential for further improvements, constantly enhanced by new research results. For more reliable quantification is also possible to implement complex algorithms based on FFT signal processing, which are independent on amplitude variations. The new designed system can be operated by standard PC and daily used smart-phones systems (Android and iOS system) with high computing power. The special electrode design will be enhanced by multiplexed impedance measurement mode in close future. New version design also includes acceleration and high-resolution temperature sensors.
Acknowledgement
Presented work was supported by the projects APVV-0496-12, VEGA 1/0459/12 and VEGA 1/1110/12.
Ing. Erik Vavrinský, Ph.D.
Institute of Electronics and Photonics
Slovak University of Technology,
Ilkovicova 3, 812 19 Bratislava, Slovakia
E-mail: erik.vavrinsky@stuba.sk
Phone: +421 (2) 60 291 65
Sources
[1] Nitish, V. Thakor. Bipotentials and Electrophysiology Measurement, CRC Press LLC. http://www.engnetbase.com, 2000.
[2] Merlo, A., Campanini, I. Technical Aspects of Surface Electromyography for Clinicians, The Open Rehabilitation Journal, 2010, 3, 98-109.
[3] Konrad, P. The ABC of EMG - A Practical Introduction to Kinesiological Electromyography, Noraxon INC. USA, 2005.
[4] Lee, K. S. EMG-Based Speech Recognition Using Hidden Markov Models With Global Control Variables, IEEE Transactions on Biomedical Engineering, Vol. 55, No. 3, 2008, pp. 930-940, ISSN 0018-9294.
[5] Merlo, A., Farina, D., Merletti, R. A Fast and Reliable Technique for Muscle Activity Detection From Surface EMG Signals, Transactions On Biomedical Engineering, Vol. 50, No. 3, 2003, pp. 316-323, ISSN 0018-9294.
[6] De Luca, C. J. Electromyography.Encyclopaedia of Medical Devices and Instrumentation. (John G. Webster Ed.) John Wiley Publisher; 2006, p. 98–109.
[7] Stegemana, D. F., Bloka, J. H., Hermensb, H. J., Roeleveldd, K. Surface EMG models: properties and applications. Journal of Electromyography and Kinesiology, Volume 10, Issue 5; 2000, p. 313–326.
[8] Quasthoff, S. Grundlagen der EMG Untersuchung, Das Neurophysiologie-Labor. Volume 32, Issue 1, 2010; p. 1–27, ISSN 1439-4847, 10.1016/j.neulab.2009.08.003.
[9] Kamen, G, Gabriel D. A. Essentials of electromyography, Champaign, IL, Human Kinetics; 2010. p. 156–158.
[10] Huhta, J. C., Webster, J.G. “60-Hz interference in electrocardiography,” IEEE Trans. Biomed. Eng., 20, 91–101, 1973.
[11] Kugelstadt, T. Getting the most out of your instrumentation amplifier design. http://www.ti.com/; 2005.
[12] Kitchin, Ch., Counts L. A Designer’s guide to instrumentation amplifiers 3RD Edition. http://www.analog.com/; 2006.
[13] Abad, S. L. M, Maghooli, K. Low supply voltage electrocardiogram signal amplifier. IEEE; 2007, p. 799-801.
[14] Texas Instruments. OPA2335 Datasheet. http://www.ti.com/; 2003.
[15] Vavrinsky, E., Rendek, K., Daricek, M., Donoval, M., Horinek, F., Horniak, M., Donoval, D. Electrode configuration for EMG measurements. In: ASDAM 2010. The Eighth International Conference on Advanced Semiconductor Devices and Microsystems.Smolenice, Piscataway: Institute of Electrical and Electronics Engineers; 2010, ISBN 978-1-4244-8572-7.
[16] Ray, G., Guha, S. Relationship between the surface e.m.g. and muscular force, Medical and Biological Engineering and Computing. Volume: 21, Issue: 5, Springer Berlin / Heidelberg; 1983, ISSN 0140-0118, p. 579-586, Doi: 10.1007/BF02442383.
[17] Enoka, R. M. Muscle fatigue – from motor units to clinical symptom, Journal of Biomechanics, Volume 45, Issue 3; 2012, p. 427–433.
[18] Calvert, T. W, Chapman, A. E. The Relationship Between the surface EMG and Force Transients in Muscle: Simulation and Experimental Studies, Proceedings of the IEEE, VOL 65, NO.5; 1977.
[19] Labchart. ADInstruments, http://www.adinstruments.com/, 2012.
Labels
BiomedicineArticle was published in
The Clinician and Technology Journal
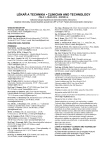
2014 Issue 3
Most read in this issue
- Vliv průtočného odporu dýchacích cest a alveolární poddajnosti na dechový objem při vysokofrekvenční oscilační ventilaci
- Health Technology Assessment for Medical Devices
- Monitoring of EMG to force ratio using new designed precise wireless sensor system
- Remote Pulse Oximetry Imaging – Fundamentals and Applications