Perspectives on pharmacological and clinical benefits from sirtuin 1 activators in oxidative damage
Authors:
Nikolina Kutinová Canová; Norbert Gaier; Hassan Farghali
Authors‘ workplace:
Karlova Univerzita v Praze, 1. lékařská fakulta, Farmakologický ústav
Published in:
Čas. Lék. čes. 2012; 151: 187-189
Category:
Review Articles
Overview
Sirtuins, named after their homology to the Saccharomyces cerevisiae Silent Information Regulator Two, constitute a family of highly conserved nicotinamide adenine dinucleotide-dependent enzymes that deacetylate histones and residues of acetylated lysine. The main aim of this article is to put forward the pharmacological importance of major sirtuin 1 activators of natural or synthetic origin tested in case of oxidative damage during the last years. The related bioactivity of these activators as “lead” compounds in the search for new drugs and medicines is also described. With recent development about the cross talks between sirtuin 1 and its modulators (e.g. resveratrol), pharmacological and clinical research on this topic is getting new horizon.
Key words:
antioxidants; caloric restriction; longevity; metabolic diseases; nutraceuticals; oxidative stress; polyphenols; resveratrol; sirtuins; SIRT1 activators.
BACKGROUND
Oxidative damage is known to be associated with the aging process and various chronic diseases as diabetes mellitus, atherosclerosis, cataract, macular degeneration, and Alzheimer disease. Oxidative damage may be either a cause or an effect; therefore, its accurate evaluation using specific biomarkers is needed. When oxidative damage acts as a causative factor of disease, its biomarkers can be a useful tool to better understand the pathogenic mechanisms involved, find novel drug targets, and evaluate effective defense strategies such as pharmaceuticals, nutraceuticals, or food and derivatives. Generally, oxidative stress arises when the production of reactive oxygen species overwhelms the intrinsic anti-oxidant defenses. Reactive oxygen species play important roles as second messengers in many intracellular signaling cascades aimed at maintaining the cell in homeostasis with its immediate environment. At higher levels, however, they can cause indiscriminate damage to biological molecules, leading to loss of function and even cell death.
There are several thousands of published reports that deal with antioxidants in health and disease with promising, sometimes conflicting results which may lead to a kind of confusion about applications of antioxidants (1). Nevertheless, there is growing scientific agreement that antioxidants, particularly the polyphenolic forms, may help lower the incidence of disease, such as certain cancers, cardiovascular and neurodegenerative diseases, DNA damage, or even have anti-aging properties. On the other hand, questions remain as to whether some antioxidants or phytochemicals potentially could do more harm than good, as an increase in glycation-mediated protein damage (carbonyl stress) and some risk has been reported. Nevertheless, the quest for healthy aging has led to the use of antioxidants as a means to disrupt age-associated deterioration in physiological function, dysregulated metabolic processes or prevention of many age-related diseases. Although a diet rich in polyphenolic forms of antioxidants does seem to offer hope in delaying the onset of age-related disorders, it is still too early to define their exact clinical benefit for treating age-related disease.
In fact, the natural products from plants and microorganisms traditionally have provided the pharmaceutical industry with important sources of "lead" compounds in the search for new drugs and medicines where the diversity of polyphenol structure is huge. The most important polyphenols in our food are being phenolic acids and flavonoids. Several health beneficial activities have been attributed to these naturally occurring plant flavonols, including antioxidative, anticarcinogenic, vasoprotective, anti-inflammatory, neuroregenerative, antidiabetic, antiplatelet activities, among which antioxidant properties remain the main topic investigated in recent years (2). The flavonoid silymarin and silibinin are substances with documented cytoprotective properties. Their mechanisms of action are still poorly understood and various explanations were provided. Other naturally occurring substances of plant origin have been claimed to possess cytoprotective actions include curcumin, tetrahydrocurcumin, catechin, quercetin and beta-carotene. Other naturally occurring cytoprotective agent is resveratrol (trans-3,4’,5-trihydroxystilbene) which is also a polyphenolic compound found in plant sources belonging to the stilbene class. Resveratrol has been detected in trees, in a few flowering plants, in peanuts, and in grapevines but the major dietary sources of resveratrol include grapes, wine, peanuts, and peanut products. The protective role of resveratrol in a number of tissues was reported (3-6). For resveratrol, we have found that among the mechanisms responsible for the an effective hepatoprotective effect in the experimental fulminant hepatic failure are reduction in nitric oxide production, downregulation of inducible nitric oxide synthase, modification of other oxidative stress parameters and modulation of heme oxygenase-1 which led to overall improvement in hepatotoxic markers and morphology after the hepatic insult (7). One of the suggested mechanisms of the beneficial effects of resveratrol is due to the activation of sirtuin 1 (silent information regulator T1 or SIRT1).
SIRTUIN 1 FUNCTIONS
Sirtuins, named after their homology to the Saccharomyces cerevisiae silent information regulation 2 (Sir2) protein, constitute a family of highly conserved NAD+-dependent enzymes that deacetylate histones and residues of acetylated lysine (8). A common feature of the activity of sirtuins is their dependence on intracellular levels of NAD (nicotinamide adenine dinucleotide) in its oxidized (NAD+) or reduced form (NADH). Sirtuins are reported to act as sensors that detect cellular energy availability (9). Calorie restriction extends lifespan in organisms ranging from yeast to mammals. In yeast, the Sir2 gene mediates the life-extending effects of calorie restriction (10). It is shown that the mammalian Sir2 orthologue, SIRT1, activates a critical component of calorie restriction in mammals; that is, fat mobilization in white adipocytes (11). Recent studies suggest a key role for the mammalian SIRT1 in the adequate cellular response to metabolic stress events such as nutrient overload or nutrient deprivation and that SIRT1 and SIRT1 activators play role in the protection from detrimental effects of metabolic stressors (12). The key role in the regulation of adipogenesis is played by nuclear receptor PPAR-γ (peroxisome proliferator-activated receptor-γ). Indeed, upon food withdrawal SIRT1 protein binds to and represses genes controlled by the fat regulator PPAR-γ, including genes mediating fat storage (11). SIRT1 represses PPAR-γ by docking with its cofactors. Repression of PPAR-γ by SIRT1 is also evident in 3T3-L1 adipocytes, where overexpression of SIRT1 attenuates adipogenesis, and RNA interference of SIRT1 enhances it. Mobilization of fatty acids from white adipocytes upon fasting is compromised in SIRT1 (+/-) mice. In differentiated fat cells, up-regulation of SIRT1 triggers lipolysis and loss of fat. As result, a reduction in fat is sufficient to extend murine lifespan (10). The above-mentioned results provide a possible molecular pathway connecting calorie restriction to life extension in mammals.
In a study using liver-specific SIRT1-knockout mice, Chen et al. (13) challenge the assumption that calorie restriction always activates SIRT1 in all tissue types by demonstrating that SIRT1 activity is reduced in the liver during calorie restriction yet activated when mice are fed a high calorie diet. The study determines that liver-specific SIRT1-knockout mice have at least some protection, compared with wild-type mice, from accumulating fat while on a high-calorie ‘western’ diet. In contrast, while under calorie restriction, the liver-specific knockout mice have the same phenotype as wild-type mice. These observations suggest that hepatic SIRT1 may be inactivated during calorie restriction in normal mice and activated while on a high-calorie diet, opposite to what occurs in the muscle and the white adipose tissue that can be explained by different redox status and NAD+/NADH ratio in the liver from other tissues during studied conditions. The authors raised a possibility that SIRT1 inhibitors specifically targeted to the liver may be of benefit in treating obesity. Indeed, SIRT1 acts as cellular energy sensor that links metabolic stressors with an adequate cellular response. In the liver, SIRT1 activates PGC-1α (PPAR-γ coactivator-1α) during fasting to induce gluconeogenesis (13).
Recent evidence demonstrates that there are similarities between AMPK (adenosine 5´-monophosphate-activated protein kinase) and SIRT1. They have similar effects on diverse processes such as cellular fuel metabolism, inflammation, and mitochondrial function. These similarities occur because AMPK and SIRT1 both regulate each other and share many common target molecules (14). These findings and the concurrent demonstration by many laboratories of common activators, actions, and target molecules of SIRT1 and AMPK led to an examination of a possible linkage between SIRT1 and the primary upstream AMPK kinase, liver kinase B1. For example, the evidence for a SIRT1/liver kinase B1/AMPK signaling mechanism was obtained by Hou et al. (15), who demonstrated that the ability of polyphenols (resveratrol, SI 17834) to activate AMPK in cultured HepG2 cells and mouse liver in vivo required the presence of both SIRT1 and liver kinase B1.
Another important target for SIRT1 is NF-κB (nuclear factor-kappa B), a regulator of many processes, including the cell cycle, apoptosis, and inflammation. SIRT1 downregulates NF-κB-mediated proinflammatory effects by deacetylating the RelA/p65 subunit of NF-κB (16,17). It is well-documented that chronic overfeeding, by increasing circulating fatty acids, might lead to inflammation, insulin resistance and injury in the liver. SIRT1-overexpressing transgenic mice have decreased hepatic NF-κB activity, which protects from high-fat diet/lipid-induced hepatic inflammation, glucose intolerance, and nonalcoholic fatty liver disease (18). Another study finds that SIRT1-mediated attenuation of NF-κB signaling prevents cytokine-induced pancreatic β-cell damage (19).
SIRTUIN 1 ACTIVATION
Milne et al. (20) identified novel small-molecule SIRT1 activators with 1000-fold higher pharmacologic potency compared with the structurally unrelated polyphenolic SIRT1 activator, resveratrol. Obese rodent models treated with these SIRT1 activators show improved whole-body glucose homeostasis and insulin sensitivity in liver, skeletal muscle, and adipose tissue, as well as an increased mitochondrial capacity in skeletal muscle. A very potent SIRT1 agonist, SRT1720, further increases endurance and enhances oxidative metabolism in muscle, liver, and brown adipose tissue, which might contribute to the observed protection from diet-induced obesity (12). Such increased metabolic performance might be induced by concerted deacetylation of the SIRT1 substrates PGC-1α, FOXO-1 (forkhead box-containing protein-1), and p53. SIRT1-mediated, indirect activation of AMPK and acetyl-CoA carboxylase might also contribute to the observed protection from nonalcoholic fatty liver disease in SRT1720-treated mice fed a high-fat diet (20). These interesting reports on small-molecule SIRT1 activators also suggest promising new therapeutic approaches to treat metabolic diseases such as type 2 diabetes or nonalcoholic fatty liver disease (12,20).
Resveratrol is the most widely investigated activator of SIRT1 as a small polyphenol that improves insulin sensitivity and vascular function, boosts endurance, inhibits tumor formation, and ameliorates the early mortality associated with obesity in mice. Most of these effects are consistent with the modulation of SIRT1 targets, such as PGC-1α and NF-κB. Moreover, it can also activate AMPK, inhibit cyclooxygenases, and influence a variety of other enzymes (8). The novel activator, SRT1720, as well as various methods to manipulate NAD+ metabolism (e.g. resveratrol-induced AMPK's elevation of NAD levels in recovering and spared cells), are emerging as alternative methods to increase SIRT1 activity, and in many cases recapitulate effects of resveratrol.
CURRENT AND FUTURE RESEARCH ON SIRTUIN 1 ACTIVATION AND ITS CLINICAL POTENTIAL
This article is focusing on the pharmacological importance of major SIRT1 activators of natural or synthetic origin tested during the last years and their related bioactivity as lead compounds. The bioactivity of these compounds depends on various molecular biological effects and on their pharmacokinetic profiles. Nowadays, an emphasis is put on the importance of oxidative damage on the electron transport chain for the rational use of mitochondria-targeted antioxidants e.g. polyphenols. Moreover, molecules of interest of flavonoid type are thoroughly studied as highly potential lead compounds in type 2 diabetes mellitus (20). Therefore, it will be important to fully elucidate the role of SIRT1 in the effects of polyphenols, such as resveratrol, which appears to reduce oxidative stress even in the brain (21). More work is needed to address these questions.
These efforts are especially important in light of the fact that SIRT1 activators are entering clinical trials in humans, and “nutraceutical” formulations containing resveratrol are already widely available. With recent development about the cross talk between SIRT1 and its modulators, pharmacological and clinical research on this topic is getting new horizon.
Abbreviations
- AMPK - adenosine 5´-monophosphate-activated protein kinase
- NAD+ - nicotinamide adenine dinucleotide - oxidized form
- NF-κB - nuclear factor-kappa B
- PGC-1α - peroxisome proliferator-activated receptor-gamma coactivator-1alpha
- PPAR-γ - peroxisome proliferator-activated receptor-gamma
- Sir2 - silent information regulator 2 from Saccharomyces cerevisiae
- SIRT1 - silent information regulator T1 or sirtuin 1
This work was supported by the research grants: VZ MSM 0021620807, GACR 305/09/0004, GACR 305/07/0061, PRVOUK-P25/LF1/2.
Corresponding address:
MUDr. Nikolina Kutinová Canová, Ph.D.
Charles University in Prague, First Faculty of Medicine, Institute of Pharmacology
Albertov 4, 120 00 Prague 2
e-mail: ncano@lf1.cuni.cz
phone/fax: +420 224968104
Sources
1. Obrenovich ME, Li Y, Parvathaneni K, et al. Antioxidants in health, disease and aging. CNS Neurol Disord Drug Targets 2011; 10: 192–207.
2. Survay NS, Upadhyaya CP, Kumar B, et al. New genera of flavonols and flavonol derivatives as therapeutic molecules. J Korean Soc Appl Biol Chem 2011; 54: 1–18.
3. Hebbar V, Shen GX, Hu R, et al. Toxicogenomics of resveratrol in rat liver. Life Sci 2005; 76: 2299–2314.
4. Ara C, Kirimlioglu H, Karabulut AB, et al. Protective effect of resveratrol against oxidative stress in cholestasis. J Surg Res 2005; 127: 112–117.
5. Yang H, Lee MK, Kim YC. Protective activities of stilbene glycosides from Acer mono leaves against H2O2-induced oxidative damage in primary cultured rat hepatocytes J Agric Food Chem 2005; 53: 4182–4186.
6. Wu SL, Pan CE, Yu L, Meng KW. Immunosuppression by combined use of cyclosporine and resveratrol in a rat liver transplantation model. Transplant Proc 2005; 37: 2354–2359.
7. Farghali H, Černý D., Kameníková L, et al. Resveratrol attenuates lipopolysaccharide-induced hepatitis in D-galactosamine sensitized rats: Role of nitric oxide synthase 2 and heme oxygenase-1. Nitric Oxide 2009; 21: 216–225.
8. Baur JA. Biochemical effects of SIRT1 activators. Biochim Biophys Acta 2010; 1804: 1626–1634.
9. Chaudhary N, Pfluger PT. Metabolic benefits from Sirt1 and Sirt1 activators. Curr Opin Clin Nutr Metab Care 2009; 12: 431–437.
10. Lin SJ, Ford E, Haigis M, et al. Calorie restriction extends yeast life span by lowering the level of NADH. Genes Dev 2004; 18: 12–16.
11. Picard F, Kurtev M, Chung NJ, et al. Sirt1 promotes fat mobilization in white adipocytes by repressing PPAR-gamma. Nature 2004; 429: 771–776.
12. Feige JN, Lagouge M, Canto C, et al. Specific SIRT1 activation mimics low energy levels and protects against diet-induced metabolic disorders by enhancing fat oxidation. Cell Metab 2008; 8: 347–358.
13. Chen D, Bruno J, Easlon E, et al. Tissue-specific regulation of SIRT1 by calorie restriction. Genes Dev 2008; 22: 1753–1757.
14. Ruderman NB, Xu XJ, Nelson L, et al. AMPK and SIRT1: a long-standing partnership? Am J Physiol Endocrinol Metab 2010; 298: E751–E760.
15. Hou X, Xu S, Maitland-Toolan KA, et al. SIRT1 regulates hepatocyte lipid metabolism through activating AMP-activated protein kinase. J Biol Chem 2008; 283: 20015–20026.
16. Yang SR, Wright J, Bauter M, et al. Sirtuin regulates cigarette smoke-induced proinflammatory mediator release via RelA/p65 NF-kappaB in macrophages in vitro and in rat lungs in vivo: implications for chronic inflammation and aging. Am J Physiol Lung Cell Mol Physiol. 2007; 292: L567–L576.
17. Yeung F, Hoberg JE, Ramsey CS, et al. Modulation of NF-kappaB-dependent transcription and cell survival by the SIRT1 deacetylase. EMBO J 2004; 23: 2369–2380
18. Pfluger PT, Herranz D, Velasco-Miguel S, et al. Sirt1 protects against high-fat diet-induced metabolic damage. Proc Natl Acad Sci USA 2008; 105: 9793–9798.
19. Lee JH, Song MY, Song EK, et al. Overexpression of SIRT1 protects pancreatic beta-cells against cytokine toxicity by suppressing the nuclear factor-kappaB signaling pathway. Diabetes 2009; 58: 344–351.
20. Milne JC, Lambert PD, Schenk S, et al. Small molecule activators of SIRT1 as therapeutics for the treatment of type 2 diabetes. Nature 2007; 450: 712–716.
21. Venturini CD, Merlo S, Souto AA, et al. Resveratrol and red wine function as antioxidants in the nervous system without cellular proliferative effects during experimental diabetes. Oxid Med Cell Longev 2010; 3: 434–441.
Labels
Addictology Allergology and clinical immunology Anaesthesiology, Resuscitation and Inten Angiology Audiology Clinical biochemistry Dermatology & STDs Paediatric dermatology & STDs Paediatric gastroenterology Paediatric gynaecology Paediatric surgery Paediatric cardiology Paediatric nephrology Paediatric neurology Paediatric clinical oncology Paediatric ENT Paediatric pneumology Paediatric psychiatry Paediatric radiology Paediatric rheumatology Paediatric urologist Diabetology Endocrinology Pharmacy Clinical pharmacology Physiotherapist, university degree Gastroenterology and hepatology Medical genetics Geriatrics Gynaecology and obstetrics Haematology Hygiene and epidemiology Hyperbaric medicine Vascular surgery Chest surgery Plastic surgery Surgery Medical virology Intensive Care Medicine Cardiac surgery Cardiology Clinical speech therapy Clinical microbiology Nephrology Neonatology Neurosurgery Neurology Nuclear medicine Nutritive therapist Obesitology Ophthalmology Clinical oncology Orthodontics Orthopaedics ENT (Otorhinolaryngology) Anatomical pathology Paediatrics Pneumology and ftiseology Burns medicine Medical assessment General practitioner for children and adolescents Orthopaedic prosthetics Clinical psychology Radiodiagnostics Radiotherapy Rehabilitation Reproduction medicine Rheumatology Nurse Sexuology Forensic medical examiner Dental medicine Sports medicine Toxicology Traumatology Trauma surgery Urology Laboratory Home nurse Phoniatrics Pain management Health Care Medical studentArticle was published in
Journal of Czech Physicians
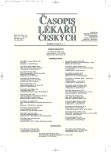
Most read in this issue
- Cystic polyposis of the stomach (fundic gland polyps) – relationship to the absence of Helicobacter pylori infection and a therapy with drugs suppressing gastric acidity
- Electronic cigarette
- Determination of needs of terminally ill patients in the hospice care
- Perspectives on pharmacological and clinical benefits from sirtuin 1 activators in oxidative damage