Mice lacking individual molecular forms of cholinesterases
Authors:
Matej Kučera; Anna Hrabovská
Published in:
Čes. slov. Farm., 2016; 65, 52-63
Category:
Review Articles
Overview
Acetylcholinesterase (AChE) and butyrylcholinesterase (BChE) represent a small family of enzymes called cholinesterases. These enzymes are in the organisms either soluble or anchored through anchoring proteins collagen Q (ColQ) and proline-rich membrane anchor (PRiMA). Knowledge of molecular biology and genetics of cholinesterase and their anchoring proteins resulted in the preparation of mutant mice with the absence of different molecular forms of cholinesterases. So far a number of mutant mice were prepared with a genetic modification on the genes encoding cholinesterases or anchoring proteins. The mice with mutation in the genes encoding the cholinesterases are: the mice with the absence of AChE, mice with the absence of BChE, mice with a deletion of exon 5 and 6 in the AChE gene and mice with the absence of AChE in muscles. The mice with a mutation in the genes encoding anchoring proteins include the mice with the absence of AChE and BChE anchored by ColQ and mice with the absence of AChE and BChE anchored by PRiMA. The study of adaptation changes results from the absence of cholinesterases led to the enrichment of existing knowledge about cholinesterases and the cholinergic nervous system.
Key words:
absence of cholinesterases • mutant mice • acetylcholinesterase • butyrylcholinesterase
Sources
1. Massoulié J., Pezzementi L., Bon S., Krejci E., Vallette F. M. Molecular and cellular biology of cholinesterases. Prog. Neurobiol. 1993; 41(1), 31–91.
2. Hrabovská A. Localization, processing and function of cholinesterases in striatum. In: Striatum: anatomy, functions and role in disease. 1. vydanie. New York: Nova Sciene Publishers 2012; 1–36.
3. Hrabovská A. Krejci E. Reassessment of the role of the central cholinergic system. J. Mol. Neurosci. 2014; 53(3), 352–358.
4. Masson P., Lockridge O. Butyrylcholinesterase for protection from organophosphorus poisons: catalytic complexities and hysteretic behavior. Arch. Biochem. Biophys. 2010; 494(2), 107–120.
5. Hrabovská A., Debouzy J. C., Froment M. T., Devínsky F., Pauliková I., Masson P. Rat butyrylcholinesterase-catalysed hydrolysis of N-alkyl homologues of benzoylcholine. FEBS J. 2006; 273(6), 1185–1197.
6. Appleyard M. E., McDonald B. Acetylcholinesterase and butyrylcholinesterase activities in cerebrospinal fluid from different levels of the neuraxis of patients with dementia of Alzheimer type. J. Neurol. Neurosurg. Psychiatry 1992; 55(11), 1074–1078.
7. Kálmán J., Juhász A., Janka Z., Rakonczay Z., Abrahám G., Boda K., Farkas T., Penke B. Serum butyrylcholinesterase activity in hyperlipidaemia. Atheroslerosis 2004; 173(1), 145–146.
8. Manoharan I., Boopathy R., Darvesh S., Lockridge O. A medical health report on individuals with silent butyrylcholinesterase in the Vysya community of India. Clin. Chim. Acta. 2007; 378(1–2), 128–135.
9. Getman G. T., Eubanks J. H., Camp S., Evans G. A., Taylor P. The human gene encoding acetylcholinesterase is located on the long arm of chromosome 7. Am. J. Hum. Genet. 1992; 51(1), 170–177.
10. Arpagaus M., Kott M., Vatsis K. P., Bartels C. F., La Du B. N., Lockridge O. Structure of the gene for human butyrylcholinesterase. Evidence for a single copy. Biochemistry 1990; 29(1), 124–131.
11. Massoulié J. The origin of the molecular diversity and functional anchoring of cholinesterases. Neurosignals 2002; 11, 130–143.
12. Grisaru D., Deutsch V., Shapira M., Pick M., Sternfeld M., Melamed-Book N., Galyam N., Gait M. J., Owen D., Lessing J. B., Eldor A., Soreq H. ARP, a peptide derived from the stress-associated acetylcholinesterase variant, has hematopoietic growth promoting activities. Mol. Med. 2006; 7(2), 93–105.
13. Zimmerman G., Soreq H. Readthrough acetylcholinesterase: a multifaceted inducer of stress reactions. J. Mol. Neurosci. 2006; 30(1–2), 197–200.
14. Härtl R., Gleinich A., Zimmerman M. Dramatic increase in readthrough acetylcholinesterase in a cellular model of oxidative stress. J. Neurochem. 2011; 116(6), 1088–1096.
15. Dori A., Ifergane G., Saar-Levy T., Bersudsky M., Mor I., Soreq H., Wirguin I. Readthrough acetylcholinesterase in inflammation-associated neuropathies. Life Sci. 2007; 80(24–25), 2369–2374.
16. García-Ayllón M. S., Millán C., Serra-Basante C., Bataller R., Sáez-Valero J. Readthrough acetylcholinesterase is increased in human liver cirhosis. PLoS One 2012; 7(9), e44598.
17. Pegan K., Matkovic U., Mars T., Mis K., Pirkmajer S., Brecelj J., Grubic Z. Acetylcholinesterase is involved in apoptosis in the precursors of human muscle regeneration. Chem. Biol. Interact. 2010; 187(1–3), 96–100.
18. Berson A., Knobloch M., Hanan M., Diamant S., Sharoni M., Schippli D., Geyer B. C., Ravid R., Mor T. S:, Nitsch R. M., Soreq H. Changes in readthrough acetylcholinesterase expression modulate amyloid-beta pathology. Brain 2008; 131(Pt 1), 109–119.
19. Li Y., Camp S., Rachinsky T. L., Getman D., Taylor P. Gene structure of mammalian acetylcholinesterase. Alternative exons dictate tissue-specific expression. J. Biol. Chem. 1991; 266(34), 23083–23090.
20. Montenegro M. F., Ruiz-Espejo F., Campoy F. J., Munoz-Delgado E., de la Cadena M. P., Rodríguez-Berrocal F. J., Vidal C J. Cholinesterases are down-expressed in human colorectal carcinoma. Cell. Mol. Life Sci. 2006; 63(18), 2175–2182.
21. Montenegro M. F., Nieto-Cerón S., Cabezas-Herrera J., Munoz-Delgado E., Campoy F. J., Vidal C. J. Most acetylcholinesterase activity of non-nervous tissues and cells arises from the AChE-H transcript. J. Mol. Neurosci. 2014; 53(3), 429–435.
22. Moral-Naranjo M. T., Montenegro M. F., Munoz-Delgado E., Campoy F. J., Vidal C. The levels of both lipid rafts and raft-located acetylcholinesterase dimers increase in muscle of mice with muscular dystrophy by merosin deficiency. Biochim. Biophys. Acta. 2010; 1802(9), 754–764.
23. Rosenberry T. L., Roberts W. L., Haas R. Glycolipid membrane-binding domain of human erythrocyte acetylcholinesterase. Fed. Proc. 1986; 45(13), 2970–2975.
24. Massoulié J., Anselmet A., Bon S., Krejci E., Legay C., Morel N., Simon S. Acetylcholinesterase: C-terminal domains, molecular forms and functional localization. J. Physiol. Paris. 1998; 92(3–4), 183–190.
25. Massoulié J., Bon S., Perrier N., Falasca C. The C-terminal peptides of acetylcholinesterase: cellular trafficking, oligomerization and functional anchoring. Chem. Biol. Interact. 2005; 157–158, 3–14.
26. Kučera M., Hrabovská A. Molekulové formy cholínesteráz a ich kotviace proteíny. Chemické listy 2013; 107, 695–700.
27. Feng G., Krejci E., Molgo J., Cunningham j. M., Massoulié J., Sanes J. R. Genetic Analysis of Collagen Q: Roles in Acetylcholinesterase and Butyrylcholinesterase Assembly and in Synaptic Structure and Function. J. Cell Biol. 1999; 114(6), 1349–1360.
28. Krejci E., Thomine S., Boschetti N., Legay C., Sketelj J., Massoulié J. The mammalian gene of acetylcholinesterase-associated collagen. J. Biol. Chem. 1997; 272(36), 22840–22847.
29. Krejci E., Legay C., Thomine S., Sketelj J., Massoulié J. Differences in expression of acetylcholinesterase and collagen Q control the distribution and oligomerization of the collagen-tailed forms in fast and slow muscles. J. Neurosci. 1999; 19(24), 10672–10679.
30. Sigoillot S.M., Bourgeosis F., Lambergeon M., Strochlic L., Legay C. ColQ controls postsynaptic differenciation at the neuromuscular junction. J. Neurosci. 2010; 30(1), 13–23.
31. Engel A.G., Shen X. M., Selcen D., Sine S. M. What have we learned from the congenital myasthenic syndromes. J. Mol. Neurosci. 2010; 40(1–2), 143–153.
32. Perrier A. L., Massoulié J., Krejci E. PRiMA: The Membrane Anchor of Acetycholinesterses in the Brain. Neuron. 2002; 33, 275–285.
33. Chen V. P., Xie H. Q., Chan W. K., Leung K. W., Chan G. K., Choi R. C., Bon S., Massoulié J., Tsim K W. The PRiMA-linked tetramers are assembled from homodimers: hybrid molecules composed of acetylcholinesterase and butyrylcholinesterase dimers are up-regulated during development of chicken brain. J. Biol. Chem. 2010; 285(35), 27265–27278.
34. Dobbertin A., Hrabovská A., Dembele K., Camp S., Taylor P., Krejci E., Bernard V. Targeting of acetylcholinesterase in neurons in vivo: A dual processing function for the Proline-Rich Membrane Anchor subunit and the attachment domain on the catalytic subunit. J. Neurosci. 2009; 18729(14), 4519–4530.
35. García-Ayllón M. S., Campanari M. L., Montenegro M. F., Cuchillo Ibánez I., Belbin O., Lleó A., Tsim K., Vidal C. J., Sáez-Valero J. Presenilin-1 influences processing of the acetylcholinesterase membrane anchor PRiMA. Neurobiol. Aging. 2014; 35(7), 1526–1536.
36. Dvir H., Harel M., Bon S., Liu W. Q., Vidal M., Barbay C., Sussman J. l., Massoulié J. Silman I. The synaptic acetylcholinesterase tetramer assembles around a polyproline II helix. EMBO J. 2004; 23(22), 4394–4405.
37. Simon S.,Krejci E., Massoulié J. A four-to-one association between peptide motifs: four C-terminal domains from cholinesterase assemble with one proline-rich attachment domain (PRAD) in the secretory pathway. EMBO J. 1988; 7(10), 2983–2993.
38. Xie W., Wilder P. J., Stribley J. A., Chatonnet A., Rizzino A., Taylor P., Hinrichs S. H., Lockridge O. Knockout of the acetylcholinesterase allele in the mouse. Chem. Biol. Interact. 1999; 119-120, 289–299.
39. Xie W., Stribley J. A., Chatonnet A., Wilder P. J., Rizzino A., McComb R. D., Taylor P., Hinrichs S. H., Lockridge O. Postnatal developmental delay and supersensitivity to organophosphate in gene-targeted mice lacking acetylcholinesterase. J. Pharmacol. Exp. Ther. 2000; 293(3), 896–902.
40. Sun M., Lee C. J., Shin H. S. Reduced nicotinic receptor function in sympathetic ganglia is responsible for hypothermia in the acetylcholinesterase knockout mouse. J. Physiol. 2007; 578(Pt 3), 751–764.
41. Li B., Stribley J. A., Ticu A., Xie W., Schopfer L. M., Hammond P., Brimijoin S., Hinrichs S. H., Lockridge O. Abundant tissue butyrylcholinesterase and its possible function in the acetylcholinesterase knockout mouse. J. Neurochem. 2000; 75(3), 1320–1231.
42. Adler M., Manley H. A., Purcell A. L., Deshpande S. S., Hamilton T. A., Kan R. K., Oyler G. Lockridge O., Duysen E. G., Sheridan R. E. Reduced acetylcholine receptor density, morphological remodeling, and butyrylcholinesterase activity can sustain muscle function in acetylcholinesterase knockout mice. Muscle nerve. 2004; 30(3), 317–327.
43. Hartmann J., Kiewert C., Duysen E. G., Lockridge O., Greig N. H., Klein J. Excessive hippocampal acetylcholine levels in acetylcholinesterase-deficient mice are moderated by butyrylcholinesterase activity. J. Neurochem. 2007; 100(5), 1421–1429.
44. Duysen E.G., Li B., Xie W., Schopfer L. M., Anderson R. S., Broomfield C. A., Lockridge O. Evidence for nonacetylcholinesterase targets of organophosphorus nerve agent: supersensitivity of acetylcholinesterase konckout mouse to VX lethality. J. Pharmacol. Exp. Ther. 2001; 299(2), 528–535.
45. Duysen E.G., Stribley J. A., Fry D. L., Hinrichs S. H., Lockridge O. Rescue of the acetylcholinesterase knockout mouse by feeding a liquid diet; phenotype of the adult acetylcholinesterase deficient mouse. Brain Res. Dev. Brain Res. 2002; 137(1), 43–54.
46. Gupta R. C., Patterson G. T., Dettbarn W. D. Mechanizms of toxicity and tolerance to diisopropylphosphorofluoridate at the neuromuscular junction of the rat. Toxicol. Appl. Pharmacol. 1986; 84(3), 541–550.
47. Girard E., Bernard V., Camp S., Taylor P., Krejci E., Molgó J. Remodeling of the neuromuscular junction in mice with deleted exons 5 and 6 of acetylcholinesterase. J. Mol. Neurosci. 2006; 30(1-2), 99–100.
48. Blondet B., Carpentier G., Ferry A., Chatonnet A., Courty J. Localization fo butyrylcholinesterase at the neuromuscular junction of normal and acetylcholinesterase knockout mice. J. Histochem. Cytochem. 2010; 58(12), 1075–1082.
49. Hrabovská A., Duysen E. G., Sanders J. D., Murrin L. C., Lockridge O. Delivery of human acetylcholinesterase by adeno-associated virus to the acetylcholinesterase knockout mouse. Chem. Biol. Interact. 2005; 157–158, 71–78.
50. Mesulam M.M., Guillozet A., Shaw p., Levey A., Duysen E. G., Lockridge O. Acetylcholinesterase knockouts establish central cholinergic pathways and can use butyrylcholinesterase to hydrolyze acetylcholine. Neuroscience 2002; 110(4), 627–639.
51. Rice S. G., Nowak L., Duysen E. G., Lockridge O., Lahiri D. K., Reyes P. F. Neuropathological and immunochemical studies of brain parenchyma in acetylcholinesterase knockout mice: implications in Alzheimer’s disease. J. Alzheimers Dis. 2007; 11(4), 481–489.
52. Volpicelli-Daley L. A., Duysen E. G., Lockridge O., Levey A. I. Altered hippocampal muscarinic receptors on acetylcholinesterase-deficient mice. Ann. Neurol. 2003; 53(6), 788–796.
53. Volpicelli-Daley L. A., Hrabovská A., Duysen E. G., Ferguson S. M., Blakely R. D., Lockridge O., Levey A. I. Altered striatal function and muscarinic cholinergic receptors in acetylcholinesterase knockout mice. Mol. Pharmacol. 2003; 64(6), 1309–1316.
54. Bernard V., Brana C., Liste I., Lockridge O., Bloch B. Dramatic depletion of cell surface m2 muscarinic receptor due to limited delivery from intracytoplasmic stores in neurons of acetylcholinesterase-deficient mice. Mol. Cell Neurosci. 2003; 23(1), 121–133.
55. Li B., Duysen E. G., Volpicelli-Daley L. A., Levey A. I. Lockridge O. Regulation of muscarinic acetylcholine receptor function in acetylcholinesterase knockout mice. Pharmacol. Biochem. Behav. 2003; 74(4), 977–986.
56. Chatonnet F., Boudinot E., Chatonnet A., Taysse L., Daulon S., Champagnat J., Foutz A. S. Respiratory survival mechanisms in acetylcholinesterase knockout mouse. Eur. J. Neurosci. 2003; 18(6), 1419–1427.
57. Hrabovská A., Farár V., Bernard V., Duysen E. G., Brabec J., Lockridge O., Mysliveček J. Drastic decrease in dopaminergic receptor levels in the striatum of acetylcholinesterase knock-out mouse. Chem. Biol. Interact. 2010; 183, 1, 194–201.
58. Mysliveček J., Duysen E. G., Lockridge O. Adaptation to excess acetylcholine by downregulation of adrenoceptors and muscarinic receptors in lungs of acetylcholinesterase knockout mice. Naunyn Schmiedebergs Arch. Pharmacol. 2007; 376(1–2), 83–92.
59. Farar V., Mohr F., Legrand M., Lamotte d´Inchamps B., Leroy J., Abitbol M., Bernard V., Baud F., Fournet V., Houze P., Klein J., Plaud B., Tuma J., Zimmermann M., Acher P., Hrabovská A., Mysliveček J., Krejci E. Near-complete adaptation of the PRiMA knockout to the lack of central acetylcholinesterase. J. Neurochem. 2012; 122(5), 1065–1080.
60. Camp S., Zhang L., Marquez M., de la Torre B., Long J. M., Bucht G., Taylor P. Acetylcholinesterase (AChE) gene modification in transgenic animals: functional consequences of selected exon and regulatory region deletion. Chem. Biol. Interact. 2005; 157–158, 79–86.
61. Li B., Duysen E. G., Saunders T. L., Lockridge O. Production of the butyrylcholinesterase knockout mouse. J. Mol. Neurosci. 2006; 30(1–2), 193–195.
62. Duysen E. G., Li B., Darvesh S., Lockridge O. Sensitivity of butyrylcholinesterase knockout mice to (-)-huperzine A and donepezil suggest humans with butyrylcholinesterase deficiency may not tolerate these Alzheimer´s disease drugs and indicates butyrylcholnesterase function in neurotransmission. Toxicology 2007; 233(1–2), 60–69.
63. Li B., Duysen E. G., Carlson M., Lockridge O. The butyrylcholinesterase knockout mouse as a model for human butyrylcholinesterase deficiency. J. Pharmacol. Exp. Ther. 2008; 324(3), 1146–1154.
64. Li B., Duysen E. G., Lockridge O. The butyrylcholinesterase knockout mouse is obese on a high-fat diet. Chem. Biol. Interact. 2008; 175(1–3), 88–91.
65. Rahimi Z., Ahmadi R., Vaisi-Raygani A., Rahimi Z., Bahrehmand F., Parsian A. Butyrylcholinesterase (BChE) activity is associated with the risk of preeclampsia: influence on lipid and lipoprotein metabolism and oxidative stress. J. Matern. Fetal Neonatal Med. 2013; 26(16), 1590–1594.
66. Vallianou N. G., Evangelopoulos A. A., Bountziouka V., Bonou M. S., Katsagoni C., Vogiatzakis E. D., Avgerinos P. C., Barbetseas J., Panagiotakos D. B. Association of butyrylcholinesterase with cardiometabolic risk factors among apparently healthy adults. J. Cardiovasc. Med. (Hagerstown, Md.). 2014; 15(5), 377–383.
67. Abbott C. A., Mackness M. I., Kumar S., Olukoga A. O., Gordon C., Arrol S., Bhatnagar D., Boulton A. J., Durrington P. N. Relationship between serum butyrylcholinesterase activity, hypertriglyceridaemia and insulin sensitivity in diabetes mellitus. Clin. Sci. (Lond.) 1993; 85(1), 77–81.
68. Johnson G., Moore S. W. Why has butyrylcholinesterase been retained? Structural and functional diversification in a duplicated gene. Neurochem. Int. 2012; 61(5), 783–797.
69. Lockridge O. Genetic variants of human serum cholinesterase influence metabolism of the muscle relaxant succinylcholine. Pharmacol. Ther. 1990; 47(1), 35–60.
70. Hoffman R. S., Henry G. C., Wax P. M., Weisman R. S., Howland M. A., Goldfrank L. R. Decreased plasma cholinesterase activity enhances cocaine toxicity in mice. J. Pharmacol. Exp. Ther. 1992; 263(2), 698–702.
71. Duysen E. G., Li B., Carlson M., Li Y. F., Wieseler S., Hinrichs S. H., Lockridge O. Increased hepatotoxicity and cardiac fibrosis in cocaine treated butyrylcholinesterase knockout mice. Basic Clin. Pharmacol. Toxicol. 2008; 103(6), 514–521.
72. Duysen E. G., Lockridge O. Prolonged toxic effects after cocaine challenge in butyrylcholinesterase/plasma carboxylesterase double knockout mice: a model for butyrylcholinesterase-defficent humans. Drug Metab. Dispos. 2011; 39(8), 1321–1323.
73. Camp S., Zhang L., Krejci E., Dobbertin A., Bernard V., Girard E., Duysen E. G., Lockridge O., De Jaco A., Taylor P. Contributions of selective knockout studies to understanding cholinesterase disposition and function. Chem. Biol. Interact. 2010; 187(1–3), 72–77.
74. Camp S., De Jaco A., Zhang L., Marquez M., De la Torre B., Taylor P. Acetylcholinesterase expression in muscle is specifically controlled by a promoter-selective enhancesome in the first intron. J. Neurosci. 2008; 28(10), 2459–2470.
75. Girard E., Bernard V., Camp S., Taylor P., Krejci E., Molgó J. Remodeling of the neuromuscular junction in mice with deleted exons 5 and 6 of acetylcholinesterase. J. Mol. Neurosci. 2006; 30(1–2), 99–100.
76. Boudinot E., Bernard V., Camp S., Taylor P., Champagnat J., Krejci E., Foutaz A. S. Influence of differential expression of acetylcholinesterase in brain and muscle on respiration. Respir. Physiol. Neurobiol. 2009; 165(1), 40–48.
77. Fuentes M. E., Taylor P. Control of acetylcholinesterase gene expression during myogenesis. Neuron 1993; 10(4), 679–687.
78. Boudreau-Lariviére C., Parry D. J., Jasmin B. J. Myotubes originating from single fast and slow satellite cells display similar patterns of AChE expression. Am. J. Physiol. Regul. Integr. Comp. Physiol. 2000; 278(1), 140–148.
79. Angus L. M., Chan R. Y., Jasmin B. J. Role of intronic E- and N-box motifs in the transcriptional induction of the acetylcholinesterase gene during myogenic differentiation. J. Biol. Chem. 2001; 276(20), 17603–17609.
80. Bernard V., Girard E., Hrabovská A., Camp S., Taylor P., Plaud B., Krejci E. Distinct localization of collagen Q and PRiMA forms of acetylcholinesterase at the neuromuscular junction. Mol. Cell. Neurosci. 2011; 46(1), 272–281.
81. Minic J., Molgó J., Karlsson E., Krejci E. Regulation of acetylcholine released by muscarinic receptors at the mouse neuromuscular junction depends on the activity of acetylcholinesterase. Eur. J. Neurosci. 2002; 15(2), 439–448.
82. Nguyen-Huu T., Dobbertin A., Barbier J., Minic J., Krejci E., DUvaldestin P., Molgó J. Cholinesterases and the resistance of the mouse diaphragm to the effect of tubocurarine. Anesthesiology 2005; 103(4), 788–795.
83. Ohno K., Engel A. G., Brengman J. M., Shen X. M., Heidenreich F., Vincent A., Milone M., Tan E., Demirci M., Walsh P., Nakano S., Akiguchi I. The spectrum of mutations causing end-plate acetylcholinesterase deficiency. Ann. Neurol. 2000; 47(2), 162–170.
84. Engel A. G., Ohno K., Shen X. M., Sine S. M., Congenital myasthenic syndromes: multiple molecular targets at the neuromuscular junction. Ann. N. Y. Acad. Sci. 2003; 998, 138–160.
85. Ohno K., Ito M., Kawakami Y., Ohtsuka K. Collagen Q is a key player for developing rational therapy for congenital myasthenia and for dissecting the mechanisms of anti-MuSK myasthenia gravis. J. Mol. Neurosci. 2014; 53(3), 359–361.
86. Farar V., Hrabovská A., Krejci E., Mysliveček J. Developmental adaptation of central nervous system to extremely high acetylcholine levels. PLoS One. 2013; 8(7), e68265.
Labels
Pharmacy Clinical pharmacologyArticle was published in
Czech and Slovak Pharmacy
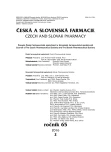
2016 Issue 2
Most read in this issue
- Oral adverse drug reactions in various medications
- Potential immunomodulatory and antiinflammatory mechanisms of probiotics
- The evaluation of the prevalence of constipation at the Centre of clinical gerontology
- Phenolic compounds of the genus Iris plants (Iridaceae)