The Far Nasal Part of the Field of Vision – Part II. Contribution to the Timely Diagnosis of Glaucoma
Authors:
J. Lešták; M. Fůs; T. Lešták; Š. Pitrová
Authors‘ workplace:
Czech Technical University in Prague, Faculty of Biomedical Engineering, Kladno, Czech Republic
Published in:
Čes. a slov. Oftal., 79, 2023, No. 6, p. 312-316
Category:
Original Article
doi:
https://doi.org/10.31348/2023/37
Overview
Aim: The aim of this study was to examine possible changes in the conventionally undiagnosed nasal visual field in patients diagnosed with early primary open angle glaucoma.
Material and Methods: Examination of the far nasal part of the visual field was performed in 30 patients (60 eyes) with early stage of primary open angle glaucoma (preperimetric stage of changes). The cohort consisted of 16 women (mean age 46.5 years) and 14 men (mean age 44.7 years). In all eyes, the glaucoma program (rapid threshold program of 50 degrees nasally and 22 degrees temporally) was performed with the Medmont M700 instrument to determine the physiological visual field. Visual acuity was 1.0 with a possible correction less than or equal to ±3 diopters and they had no other ocular defect except glaucoma disease. The visual field was subsequently examined with the same instrument by moving the fixation point 40 degrees temporally (spatially adaptive program) and simultaneously turning the head 10 degrees nasally. A total of 89 examination points were included using flicker stimuli and a range of 0–120 degrees nasally.
Results: The far nasal limit of the visual field reached 100° in 13.33% of eyes, 105° in 20% of eyes and up to 110° in 66.67% of eyes.
Conclusion: In all eyes, depression of the distal periphery of the nasal part of the visual field was found to range from 50 to 95 degrees, with a normal visual field examined by the glaucoma program.
Keywords:
primary open angle glaucoma – early diagnosis of glaucoma – far nasal visual field – early diagnosis of glaucoma
Introduction
Glaucoma is a progressive pathology in which timely diagnosis plays a fundamental role for the preservation of visual functions. With regard to the asymptomatic nature of glaucoma, timely detection of the pathology before it reaches its severe stages is demanding, and the number of diagnosed patients with glaucoma is lower than the number of undiagnosed patients [1,2]. It has been demonstrated that in glaucoma the ganglion cells of the retina (predominantly magnocellular) [3–7] wither first, followed by their retrolaminar and subsequently prelaminar located axons [8–9].
The longest used method for the diagnosis of these changes is static automatic perimetry [10]. In addition to examination of the visual field, at present diagnostic methods are used primarily to determine changes in the nerve fiber layer and the complex of ganglion cells, at the same time as determining the level of intraocular pressure. In recent years optical coherence tomography (OCT) and optical coherence tomography-angiography (OCT-A) have brought new options to the field of glaucoma diagnosis [11]. These methods are not always optimally used for verifying the early stage of glaucoma. Even when efforts are made to ensure timely diagnosis, a considerable proportion of patients with glaucoma are not detected until the advanced stage. In ophthalmological practice, perimetric examination is indicated most often for glaucomatous pathology. With regard to the fact that the finding on the optic nerve disc may conceal further neurogenic (primarily pressure) lesions, this examination remains irreplaceable upon the first contact of the ophthalmologist with the patient [12].
The majority of the textbooks on perimetry recommend testing strategies for glaucoma which examine the central part (up to 30 degrees) [13–15]. Because damage occurs above all to the ganglion cells located in the retinal periphery in glaucoma [16,17], damage to these cells logically cannot be even theoretically determined from an examination of the central part of the visual field [12]. In the nasal periphery of the retina, the density of ganglion cells is more than 300% of that in the temporal periphery, and in the upper periphery the density is 60% higher than in the lower periphery [18]. This finding should be of fundamental significance for the timely diagnosis of glaucoma. Consequently, the aim of this study was to determine whether it is possible to detect potential changes in the distal nasal part of the visual field in glaucoma with the aid of static automatic perimetry earlier than in the case of examination of the central part.
MATERIAL AND METHODS
The cohort consisted of 60 eyes in which the early stage of primary open-angle glaucoma (POAG) was verified. None of the patients was diagnosed with normotensive glaucoma. The total number of observed patients was 16 women aged 21–68 years (average age 46.5 years) and 14 men aged 21–67 years (average age 44.7 years). In addition to adequate diagnosis of glaucoma, the following inclusion criteria were stipulated: physiological ocular finding, including pupillary reaction, visual acuity of 1.0 with correction less than or equal to ±3 diopters and standard examination of the visual field (examined without artificial mydriasis by a fast threshold glaucoma program on the instrument Medmont M700, Medmont Australia) without pathological changes.
The method of testing the range of the nasal part of the visual field consisted in the use of a spatially adaptive test, in which the tested range was adapted to a total number of 89 points, with each point tested an average of 2.5 times. The minimum mutual horizontal distance between the two measured points was equal to 10°. Exposures were realized by means of the flicker method (6–18 Hz) according to the patient’s algorithm of adaptive reaction speed, without false positive or negative stimuli. The fixation point was shifted 40 degrees temporally, and the head of the examined subject turned nasally so that the range of the examined nasal part of the visual field reached 120 degrees.
RESULTS
For the sake of completeness, we must state that the Medmont instrument automatically checks fixation by means of the Heijl-Krakau method. Every tenth point is directed to the locality of a presumed blind spot, as a result of which a minor scotoma appears in the resulting tests. Shifting of the fixation point causes an erroneous presumption of the locality of the blind spot, because the program does not respect the shift of fixation. The overall defect (OD) is the central difference between the values of the age norm of the hill of vision (HoV) and the central deviation determined in the patient. This number is negative if the HoV values obtained from the patient are lower than the age norm HoV values. In our cohort the OD was in positive values in 96.7% of eyes, none of which were indicated as severe [19].
Table 1 presents the results of the perimetric examination of the nasal range of the visual field. The range of the nasal part itself was on average equal to 82.25 ± 6.72°, while in 60% of eyes the range was greater than the stated mean value. The significance of the difference of the examination result of the visual field using a conventional fast threshold program (50 degrees nasally and 22 temporally) and the extended region of testing to a total range of 120° in the same patient with primary open-angle glaucoma is demonstrated in Fig. 1.
DISCUSSION
Several studies document a normal visual field in the early stage glaucoma of 24° to 30°, but with abnormalities in the peripheral visual field. Approximately 11–17 of eyes with a diagnosis of glaucoma or glaucoma suspect and a normal central visual field manifest defects outside of the central range of 30° [20–25]. Ma et al. demonstrated glaucomatous defects in the periphery of the visual field in 18% of eyes with a normal central visual field [26]. However, of these, only measurement of the nasal periphery can contribute sufficient information to that obtained by static testing in the central 30°, in order to justify the added examination time. Nevertheless, special situations exist in which examination of the peripheral field in other or all quadrants may be useful [27]. Kerrigan-Baumrid et al. determined significant changes in the visual field in glaucoma only upon a loss of 25–35% of the retinal ganglion cells, in which the magnocellular cells were first to wither (for the examination they used a program of 0–30 degrees) [28].
Regarding the fact that hypertensive glaucoma causes greater damage to the magnocellular ganglion cells [4,29,30], examination of the peripheral part of the visual field is especially important. In our previous studies also, selective damage to the fibers of the magnocellular retinal ganglion cells was demonstrated in the case of high intraocular pressure [31,32]. In the temporal periphery of the retina there are 3x fewer ganglion cells than in the nasal part [18].
If a smaller number of ganglion cells is to cover the same surface, then their lesions will be more apparent precisely in the temporal periphery of the retina. As a result, examination of the far nasal part of the visual field should not be neglected. This study also demonstrated that in a normal visual field examined by the glaucoma program, there are large changes in the nasal distal part in the same patients.
Using the Medmont instrument, the glaucoma program examines the nasal part of the visual field up to 50 degrees and the temporal part up to 22 degrees. Upon the use of a program of the entire visual field in a range of 0–50 degrees, we did not determine any statistically significant difference either in the healthy population or in patients with glaucoma. This means that the temporal periphery of the visual field does not play such a significant role as the nasal part in glaucoma [12].
Although examination of the central part of the visual field (24–2) requires less time, which reduces patient fatigue, it has been demonstrated that in itself it identifies a commensurate amount of early diseases [33–36]. It is possible to agree with this conclusion only relatively. If the result of the examination is to assist in the determination of the diagnosis, the examination time should not be a priority.
The program we have designed for examination of the perimeter using the Medmont instrument examines the visual field in 98 points. The fast threshold glaucoma program in 104 points. Therefore it is not possible to argue with reference to the extended examination time. However, the results are incomparable.
Our cohort consisted only of patients in the early (preperimetric) stage of POAG. The results of the far nasal peripheral part of the visual field in the case of other ocular pathologies, including normotensive glaucoma, shall be in the stage of our next examination. As stated in the introduction, examination of the visual field is very important for the determination of POAG. For this reason, all patients with this diagnosis are examined not only by OCT (RNFL, vessel density), but also by perimetry.
An examination by means of static automatic perimetry ranks among the standard of all eye clinics. The method we present can therefore also represent an improvement of timely diagnosis for professionals upon first contact with a glaucoma patient.
An application for this method was submitted to the Office for Patents and Inventions under number: PV 2023-150.
CONCLUSION
The proposed method of extension of the horizontal range of the visual field by the distal part of the nasal half of the visual field in all 60 observed eyes demonstrated a depression in this area. Upon examination with the glaucoma test (fast threshold program using the Medmont instrument) in a total horizontal range of 72°, no changes of the visual field were demonstrated.
Sources
1. Burgoyne CF, Downs JC, Bellezza AJ, Suh JK, Hart RT. The optic nerve head as a biomechanical structure: a new paradigm for understanding the role of IOP-related stress and strain in the pathophysiology of glaucomatous optic nerve head damage. Prog Retin Eye Res. 2005;24:39-73.
2. Weinreb RN, Aung T, Medeiros FA. The pathophysiology and treatment of glaucoma: a review. Jama. 2014;311:1901-1911.
3. Glovinsky Y, Quigley HA, Dunkelberger GR. Retinal ganglion cell loss is size dependent in experimental glaucoma. Invest Ophthalmol Vis Sci. 1991;32:484-491.
4. Morgan JE, Uchida H, Caprioli J. Retinal ganglion cell death in experimental glaucoma. Br J Ophthalmol. 2000;84:303-310.
5. Naskar R, Wissing M, Thanos S. Detection of Early Neuron Degeneration and Accompanying Microglial Responses in the Retina of a Rat Model of Glaucoma. Invest Ophthalmol Vis Sci. 2002;43:2962-2968.
6. Shou T, Liu J, Wang W, Zhou Y, Zhao K. Differential dendritic shrinkage of alpha and beta retinal ganglion cells in cats with chronic glaucoma. Invest Ophthalmol Vis Sci. 2003;44:3005-3010.
7. Mukai R, Park DH, Okunuki Y, et al. Mouse model of ocular hypertension with retinal ganglion cell degeneration. PLoS One. 2019;14(1):e0208713. doi: 10.1371/journal.pone.0208713. eCollection 2019
8. Quigley HA, Dunkelberger GR, Green WR. Chronic human glaucoma causing selectively greater loss of large optic nerve fibers. Ophthalmology. 1988;95:357-363.
9. Soto I, Oglesby E, Buckingham BP, et al. Retinal ganglion cells downregulate gene expression and lose their axons within the optic nerve head in a mouse glaucoma model. J Neurosci. 2008;28:548-561.
10. Fankhauser F, Koch P, Roulier A. On Automation of Perimetry. Albrecht Von Graefes Arch Klin Exp Ophthalmol. 1972;184:126-150. doi:10.1007/BF02390260
11. Jia Y, Wei E, Wang X, Zhang X, et al. Optical coherence tomography angiography of optic disc perfusion in glaucoma. Ophthalmology. 2014;21:1322-1332.
12. Lestak J, Fus M. Visual Field Assessment in Hypertension Glaucoma. Cesk Slov Oftalmol. 2021;77:22-26.
13. Heijl A, Patella VM. Essential perimetry. The field analyser primer. Third edition. Carl Zeiss Meditec, Inc. 2002. ISBN 0-9721560-0-3.
14. Heijl A, Patella VM, Bengtssone B. Effective perimetry: The field analyser primer Fourth edition. Carl Zeiss Meditec, Inc. 2012. ISBN 0-9884795-0-8.
15. Racette L, Fischer M, Bebie H, Holló G, Johnson CA, Matsumoto C. A guide to perimetry and the Octopus perimeter. Haag-Streit AG Könitz, Switzerland, 2018, 7th edition, ISBN: 978-3-033-06551-2.
16. Dawson WW, Hawthorne MN, Parmer R, Hope GM, Hueter R. Very large neurons of the inner retina of humans and other mammals. Retina. 1989;9:69-74.
17. Dacey DM. Physiology, morphology and spatial densities of identified ganglion cell types in primate retina. Ciba Found Symp. 1994;184:12-28; discussion 28-34, 63-70.
18. Curcio CA, Allen KA. Topography of ganglion cells in human retina. J Comp Neurol 1990;300:5-25.
19. Uživatelská příručka. Modely M700 USB C, M700 USB CR, 5/56 Norcal Rd, Nunawading victoria 3131, Australia, Dok. č.: PN003214-DOC04 v1.8 © MEDMONT prosinec 2017.
20. Caprioli J, Spaeth GL. Static threshold examination of the peripheral nasal visual field in glaucoma. Arch Ophthalmol. 1985;103:1150-1154.
21. Seamone C, LeBlanc R, Rubillowicz M, Mann C, Orr A. The value of indices in the central and peripheral visual fields for the detection of glaucoma. Am J Ophthalmol. 1988;106:180-185.
22. Miller KN, Shields MB, Ollie AR. Automated kinetic perimetry with two peripheral isopters in glaucoma. Arch Ophthalmol. 1989;107:1316-1320.
23. Ballon BJ, Echelman DA, Shields MB, Ollie AR. Peripheral visual field testing in glaucoma by automated kinetic perimetry with the humphrey field analyzer. Arch Ophthalmol. 1992;110:1730-1732.
24. LeBlanc EP, Becker B. Peripheral nasal field defects. Am J Ophthalmol. 1971;72:415-419.
25. Odden JL, Mihailovic A, Boland MV, Friedman DS, West SK, Ramulu PY. Evaluation of Central and Peripheral Visual Field Concordance in Glaucoma. Invest Ophthalmol Vis Sci. 2016;57:2797-2804.
26. Ma X, Tang L, Chen X, Zeng L. Periphery kinetic perimetry: clinically feasible to complement central static perimetry. BMC Ophthalmol. 2021;23;21:343. doi:10.1186/s12886-021-02056-5
27. Stewart WC, Shields MB. The peripheral visual field in glaucoma: reevaluation in the age of automated perimetry. Surv Ophthalmol. 1991;36:59-69.
28. Kerrigan-Baumrind LA, Quigley HA, Pease ME, Kerrigan DF, Mitchell RS. Number of ganglion cells in glaucoma eyes compared with threshold visual field tests in the same persons. Invest Ophthalmol Vis Sci. 2000;41:741-748.
29. Morgan JE. Retinal ganglion cell shrinkage in glaucoma. J Glaucoma. 2002 Aug;11(4):365-370.
30. Shou T, Liu J, Wang W et al. Differential Dendritic Shrinkage of and ß Retinal Ganglion Cells in Cats with Chronic Glaucoma. Invest Ophthalmol Vis Sci. 2003;44: 3005-3010.
31. Lešták J, Fůs M, Král J. The Relationship Between the Thickness of cpRNFL in Segments and Intraocular Presuure. Clin Ophthalmol 2022;16:1-7.
32. Lešták J, Fůs M, Král J. Axons of retinal ganglion cells on the optic nerve disc following vessel density correction at diferent IOP values. Exp Ther Med. 25; 261,2023.
33. Aulhorn E, Karmeyer H. Frequency distribution in early glaucomatous visual field defects. Doc Ophthalmol Proc Ser. 1977;14:75-83.
34. Gramer E, Gerlach R, Krieglstein GK, Leydhecker W. Topography of early glaucomatous visual field defects in computerized perimetry. Klin Monbl Augenheilkd. 1982; 180: 515-523.
35. Heijl A, Lundqvist L. The frequency distribution of earliest glaucomatous visual field defects documented by automatic perimetry. Acta Ophthalmol (Copenh). 1984;62:658-664.
36. Heijl A. Visual field changes in early glaucoma and how to recognize them. Surv Ophthalmol. 1989;33(suppl):403-404.
Labels
OphthalmologyArticle was published in
Czech and Slovak Ophthalmology
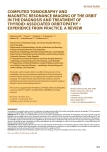
2023 Issue 6
Most read in this issue
- The Far Nasal Part of the Visual Field – Part I
- Binocular Function in Adults before and after Strabismus Surgery
- Computed tomography and magnetic resonance imaging of the orbit in the diagnosis and treatment of thyroid-associated orbitopathy – experience from practice. A Review
- Comparison of Three Methods of Tonometry in Patients with Inactive Thyroid-Associated Orbitopathy