The Far Nasal Part of the Visual Field – Part I
Authors:
J. Lešták; M. Fůs; T. Lešták; Š. Pitrová
Authors‘ workplace:
Czech Technical University in Prague, Faculty of Biomedical Engineering, Kladno, Czech Republic
Published in:
Čes. a slov. Oftal., 79, 2023, No. 6, p. 306-309
Category:
Original Article
doi:
https://doi.org/10.31348/2023/36
Overview
Aims: The aim of the study was to determine the limits of the far nasal part of the visual field.
Material and Methods: Visual field examination was performed in 15 healthy subjects (30 eyes), specifically nine women aged 20–43 years and six men aged 22–35 years. All eyes were found to have physiological ocular findings and visual acuity of 1.0 with correction less than or equal to 3 diopters. The visual field was examined with a Medmont M700 instrument by shifting the fixation point 40 degrees temporally and simultaneously turning the head nasally, with a spatial accommodation program. A total of 89 examination points were included using flicker stimuli.
Results: The far nasal limit of the visual field reached 100° in 13.3% of eyes, 105° in 20% of eyes and up to 110° in 66.7% of eyes.
Conclusion: The limit of the far nasal part of the field of vision reached 100–110 degrees (when nose shielding was eliminated).
Keywords:
visual field – nasal range of visual field – perimetry – enhanced perimetry – visual field
Introduction
In 1825, Jan Evangelista Purkyně emphasized that visual acuity in the visual field becomes increasingly less sharp in the direction towards the periphery. The peripheral parts of the retina serve mainly for orientational vision. We can determine this for example during walking, when we perceive a certain obstacle, a stone on the path, the edge of the pavement etc. with the peripheral part of the retina. Such an object is not in sharp focus, but as soon as the message from the retina reaches the cortical visual sphere, the eye reflexively turns in that direction and fixes upon the object with the central landscape [1]. Purkyně also described the limits of the visual field, specifically temporally 100 degrees, inferiorly 80 degrees, superiorly 60 degrees and the same also nasally [2]. Of the current Czech ophthalmology textbooks, mention of the range of the visual field appears only in Heissigerová et al. The visual field is the part of the space which a person perceives when fixing sight on a single point. Temporally the field has a range of 95 degrees, nasally 65, superiorly 60 and inferiorly 70 [3]. According to Pöppel and Harvey, the nasal limits of the visual field are 50 to 60 degrees, temporal up to 90 degrees [4]. Spector et al. describe peripheral vision reaching up to 100 degrees temporally and 60 degrees nasally, superiorly and inferiorly [5]. Similarly Heijl et al. and Racette et al. state the range of the visual field at more than 90 degrees temporally, 70 degrees inferiorly and 60 degrees nasally and superiorly [6–8].
Due to the inconsistency in the values of the temporal limits of the visual field, in the previous study we conducted a verification of its values with the aid of a subjective evaluation, and we then validated this result by means of a theoretical calculation. In healthy individuals we determined temporal limits of the visual field of 110 degrees [9]. We were also interested in the situation regarding the far nasal part of the visual field, which is practically unused. Even if this part appears to be “superfluous” from
a medical perspective, in our view it is diagnostically very important. The reason for this assertion is also the representation of nerve structures, which are less abundantly represented in the temporal part of the retina than in the nasal part of the retina, both on the level of the photoreceptors [10–12] and the ganglion cells of the retina [13].
This difference may be significant from a clinical perspective. For this reason, our aim in this study was also to determine the limits of the far nasal part of the visual field, i.e. exceeding 50–60 degrees. We attempted to achieve this by shifting the fixation point temporally, with simultaneous turning of the head to the other side. We did not find any similar study in the available literature.
MATERIAL AND METHODS
The cohort comprised 15 healthy subjects, specifically nine women aged 20–43 years (mean age 27.6 years) and six men aged 22–35 years (mean age 30 years). A physiological ocular finding was determined in all eyes, including pupillary reaction and visual acuity of 1.0 with correction less than or equal to 3 diopters. Because it was not possible to evaluate the accuracy of fixation, the entire cohort was composed of healthcare employees (doctors and nurses) at the JL Eye Clinic in Prague. All were familiarized with the examination by means of this technology and had repeatedly undertaken a control examination, as a result of which we were able to exclude artificial findings, including incorrect fixation. The examination was conducted under natural conditions, without artificial mydriasis.
We conducted the examination of the visual field on the instrument Medmont M700 (Medmont Pty Ltd, Australia) by means of a threshold strategy of the test, in which it was possible to supplement the nasal part of the visual field with further examination points up to a total number of 89 (spatially adaptive test). Each point was tested 2.5 times on average. Exposures to stimuli were realized by means of the flicker method (6–18 Hz), and according to the patient’s algorithm of adaptive reaction speed without false positive or negative stimuli. The fixation point was shifted 40 degrees temporally, and the head of the examined subject turned nasally so that the range of the examined nasal part of the visual field reached 110 degrees (Fig. 1 and 2).
The minimum mutual horizontal distance of the two measured points in the peripheral region was equal to 10°.
RESULTS
In all the examined eyes, a perimetric examination determined the range of the nasally seen points at 100–110 degrees. In 13.3% of eyes up to 100°, in 20% of eyes to 105° and in 66.7% of eyes up to 110° (Graph 1).
For the sake of completeness, we must state that the Medmont instrument determines fixation by means of the Heijl-Krakau method. Every tenth stimulus presupposes the position of the blind spot, as a result of which
a minor scotoma appears in the given locality. At the same time, the blind spot was erroneously localized upon shifting of the fixation point, because the program does not respect change of fixation.
DISCUSSION
To distinguish the points located below an angle of 90 degrees or larger than the fixation point, the following conditions must be met:
the optic media of the eye must receive a peripheral beam impacting on the cornea into the pupil,
the light beam reaching the retina must be sensorily processed.
Based on the geometric optics and biometric data of the measured eye, in the previous study we determined the size of the angle under which entering light beams are able to reach the posterior pole of the eye. This was attained by beams entering the eye under an angle of up to 102 degrees at a pupil width of 4.64 mm (Fig. 3) [9].
We expected to determine a similar finding also in the case of beams in the nasal half of the visual field. The corresponding peripheral parts of the retina should be sensorily functional, since 5000 rods/mm2 and approx. 500 cones/mm2 are located at 90 degrees nasally, and this number persists also at 100 degrees. In the temporal part this number is 3000 rods/mm2 and 500 cones/mm2 at 70 degrees [10]. The density of rods in the nasal half of the retina is higher than in the temporal part [11]. A similar situation applies also to the ganglion cells, of which there are 300% more in the periphery of the nasal retina than in the temporal [13].
In the previous study, in which we determined the temporal limits of the visual field at 110 degrees, similar results were attained also in the nasal direction (limits 100 to 110 degrees). This interval may be influenced by individual pupil width. Because the number of ganglion cells in the periphery of the temporal retinal is smaller, in order to cover the retina their dendritic tree must be larger than in the nasal periphery. This is a very important fact, because in the case of primary lesions of the peripheral ganglion cells of the retina, this decrease will be more evident in the nasal half of the visual field. The magnocellular ganglion cells, which are located in the peripheral part, are characterized as follows: small number (100 000), high axonal speed of conducting stimuli, relatively large receptive field, responding to rapidly flashing light stimuli, responding to objects with a low spatial frequency and high contrast sensitivity, responding to moving stimuli, not responding to colored stimuli [14]. The magnocellular ganglion cells therefore react to contrast, structure and movement in the receptive field. From this it is possible to assume their greater sensitivity in perimetric examination, in which the stimuli used are movement [15], contrast in combination with movement [16–19] and rapidly flashing light stimuli [19–21].
Unfortunately, these technologies are not used in regular practice for examining the visual field. In our case we used flashing light stimuli. Before any applicable introduction of this method into practice, it shall be necessary to resolve control of fixation upon the shift of the fixation point, and also to resolve the most suitable type of stimulation. Although we used flicker stimuli in this study, it is possible that more appropriate stimuli will be determined. It shall also naturally be necessary to verify the distal part of the visual field in a large cohort of healthy individuals. These are the limitations of our study.
An application for this method was submitted to the Office for Patents and Inventions under number: PV 2023-150.
CONCLUSION
A range of the distal nasal limits of the visual field (following adjustment of the position of the fixation point) in healthy individuals of 100 to 110 on a horizontal plane.
Sources
1. Purkyně JE. Beobachtungen u. Versuche zur Physiologie der Sinne. II. Neue Beiträge zur Kenntniss des Sehens in subjektiver Hinsicht; Reimer, Berlin 1825.
2. Kurz J. Neuro-oftalmologická diagnostika. SZN Praha 1956, s. 1018.
3. Heissigerová J a kol. Oftalmologie. Maxdorf Jessenius. 2018. ISBN 978-80-7345-580-4.
4. Pöppel E, Harvey LO Jr. Light-difference threshold and subjective brightness in the periphery of the visual field. Psychol Forsch. 1973:145-161.
5. Spector RH. In. Walker K, Hall WD and Hurst JW. Clinical Methods: The History, Physical, and Laboratory Examinations. 3rd edition. Boston: Butterworths; 1990. ISBN-10: 0-409-90077-X.
6. Heijl A, Patella VM, Bengtssone B. Effective perimetry: The field analyser primer Fourth edition. Carl Zeiss Meditec, Inc. 2012. ISBN 0-9884795-0-8
7. Heijl A, Patella VM. Essential perimetry. The field analyser primer. Third edition. Carl Zeiss Meditec, Inc. 2002. ISBN 0-9721560-0-3.
8. Racette L, Fischer M, Bebie H, Holló G, Johnson CA, Matsumoto C. A guide to perimetry and the Octopus perimeter. Haag-Streit AG Könitz, Switzerland, 2018, 7th edition, ISBN: 978-3-033-06551-2.
9. Lestak J, Lestak T, Fus M, Klimesova I. Temporal Visual Field Border. Clin Ophthalmol. 2021 Aug 3;15:3241-3246. doi: 10.2147/OPTH.S321110
10. Østerberg G. Topography of the layer of rods and cones in the human retina. Acta ophthalmol. Suppl. 1935;13:1-102.
11. Curcio CA, Sloan KR, Kalina RE, Hendrickson AE. Human photoreceptor topogtraphy. J Comp Neurol. 1990;292:497-523. doi: 10.1002/cne.902920402
12. Mustafi D, Engel AH, Palczewski K. Structure of cone photoreceptors. Prog Retin Eye Res. 2009 Jul; 28(4):289-302.
13. Curcio CA, Allen KA. Topography of ganglion cells in human retina. J Comp Neurol. 1990;300:5-25. doi: 10.1002/cne.903000103.PMID: 2229487
14. Skalicky SE. Ocular and visual physiology. Clinical application. Springer Science+Business Media Singapore Pte Ltd., Australia 2016, Chapter 8, p. 123.
15. Bosworth CF, Sample PA, Weinreb RN. Motion perception thresholds in areas of glaucomatous visual field loss. Vision Res. 1997;37:355-364.
16. Johnson CA, Samuels SJ. Screening for glaucomatous visual field loss with frequency-doubling perimetry. Invest Ophthalmol Vis Sci. 1997;38:413-425.
17. Arend KO, Plange N. Diagnostic approaches for early detection of glaucoma progression. Klin Monbl Augenheilkd. 2006;223:194-216.
18. Ferreras A, Polo V, Larrosa JM. et al. Can frequency-doubling technology and short-wavelength automated perimetries detect visual field defects before standard automated perimetry in patients with preperimetric glaucoma? J Glaucoma. 2007;16:372-383.
19. Nomoto H, Matsumoto C, Takada S. et al. Detectability of glaucomatous changes using SAP, FDT, flicker perimetry, and OCT. J Glaucoma. 2009;18:165-171.
20. Horn FK, Jonas JB, Korth M, Junemann A, Grundler A.The fullfield flicker test in early diagnosis of chronic open-angle glaucoma. Am J Ophthalmol. 1997;123:313-319.
21. Horn FK, Tornow RP, Junemann AG, Laemmer R, Kremers J. Perimetric measurements with flicker-defined form stimulation in comparison with conventional perimetry and retinal nerve fiber measurements. Invest Ophthalmol Vis Sci. 2014;55:2317-2323.
Labels
OphthalmologyArticle was published in
Czech and Slovak Ophthalmology
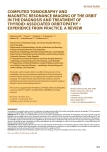
2023 Issue 6
Most read in this issue
- The Far Nasal Part of the Visual Field – Part I
- Binocular Function in Adults before and after Strabismus Surgery
- Computed tomography and magnetic resonance imaging of the orbit in the diagnosis and treatment of thyroid-associated orbitopathy – experience from practice. A Review
- Comparison of Three Methods of Tonometry in Patients with Inactive Thyroid-Associated Orbitopathy