THE EFFECT OF SPEED ON GAIT ASYMMETRY IN SUBJECT WITH CONGENITAL TIBIAL DEFICIENCY: A CASE STUDY
Authors:
Jitka Marencakova; Tomas Gryc; Frantisek Zahalka
Authors‘ workplace:
Sport research centre, Faculty of Physical Education and Sport, Charles University, Prague, Czech Republic
Published in:
Lékař a technika - Clinician and Technology No. 4, 2019, 49, 112-118
Category:
Overview
Congenital tibial deficiency is a rare developmental skeletal disorder. When the surgical treatment fails the amputation and/or prosthesis is needed. Recent studies of prosthetic gait present only data for acquired case of prosthetic gait, mainly measured in comfortable self-selected speed, which does not reflect changes visible only during higher demands conditions i.e. faster gait. Prosthetic gait of acquired cases (i.e. traumatic amputation) differs from normal gait mostly in gait asymmetry (GA). However, it was not yet studied in a developmental case of prosthetic gait. In this case study, GA changes in different speed of walking with ankle-foot prosthesis were identified for unilateral congenital tibial deficiency in a young healthy man. Selected joint kinematic, spatial-temporal, and kinetic gait parameters were collected using 3D motion capture system and treadmill with force plate simultaneously. Mean values, SD, and symmetry indexes were calculated in different walking speeds and descriptively analyzed. Results show developmental prosthetic gait specific pattern and GA in most of the measured gait parameters. Kinematic parameters of joint angular ranges register decreasing GA with increasing gait speed on intact limb side. Spatial-temporal parameters present decreasing GA with increasing speed on the prosthetic limb side for double support and step duration. Kinetic parameters show increasing GA on intact limb in all parameters, except loading rate which decrease with increasing speed. In the case of congenital tibial deficiency, gait pattern, GA and compensatory mechanisms differ to the acquired prosthetic gait and depend on walking speed. These findings complete the missing point in prosthetic gait research and may serve as a base for further research of differences between developmental and acquired cases of prosthetic gait patterns.
Klíčová slova:
chůze – Walking – Velocity
Introduction
The congenital tibial deficiency is a developmental skeletal malformation of a lower limb. This rare disorder with occurrence 1 per 1 million births is also known as congenital hemimelia, dysplasia, or aplasia of tibia and is characterized by shortening or missing of tibia bone with possible accompanying anomalies i.e. deformation of the foot, mirror foot, polydactyl occurrence, distal insertion of the quadriceps, vasculoneural anomalies, and other congenital abnormalities [1, 2]. Such developmental cases are solved surgically either by bone’s prolongation, joint reconstruction or transtibial (TT) amputation with following ankle-foot prosthetic management [1, 3, 4].
Research of acquired cases of prosthetic gait (i.e. after trauma or vascular related amputations) which are more common than developmental cases, refers that unilateral prosthetic gait is characterized by increased between limb gait asymmetry with distinct adaptive strategies of temporal-spatial, kinematic, and kinetic gait patterns
[5–11]. Furthermore, literature specifies that a gross gait asymmetry may lead even to a high metabolic cost and other debilitating factors [5].Gait with prosthesis in self-selected speed is slower in comparison with able-bodied gait and it varies according to a level and cause of amputation: lower level amputee walks faster than those with higher level of amputation [5, 12]. However, recent research investigates kinematic and kinetic parameters of prosthetic gait mainly in self-selected comfortable walking speed [11, 13–15]. This may mask real gait asymmetry and specific dynamic differences between intact and prosthetic limb which may only be seen during increased demands coupling with increased gait velocity. Unfortunately, there is a lack of studies of the prosthetic gait patterns and adaptive strategies in developmental cases of prosthetic gait as they are rare.
Thus, the aims of our case study were 1) to describe gait pattern in a developmental case of prosthetic gait―congenital tibial deficiency, and 2) to identify the gait asymmetry during different non-selected walking speeds. We hypothesized that gait asymmetry of joint kinematic, spatial-temporal, and kinetic gait parameters increase with increasing gait speed.
Methods
Study subject
One male individual (age = 26 years, body height = 176 cm, body weight = 65 kg) with partial unilateral congenital dysplasia of tibia on the right lower limb volunteered to participate in the study. In the early childhood, participant underwent surgery treatments: polydactyl removal, femoral de-rotation, and shank prolongation procedure. However, the prolongation procedure was not successful, furthermore, no trans-tibia amputation was performed. Thus, participant has worn the daily multi-axial ankle-foot prosthesis Venture 80A1 (Streifeneder ortho.production GmbH, Germany) adapted on his not fully developed foot from his 7 years of age to recent, 18 years in total. Participant regularly uses the prosthesis and has no other disabilities. He leads an active normal life with para sport activities. He is a national level para sport athlete in table tennis. Research was approved by an institutional ethics com-mittee and participant signed an informed consent.
Gait parameters
This study focused on measuring selected joint kine-matic, spatial-temporal, and kinetic gait parameters. As for joint kinematic parameters, we focused on angular range of motion of hip, knee, and ankle joint in sagittal plane. From spatial-temporal parameters, we observed step duration, double support duration, single support duration, and, step length. For investigation of kinetic of gait, we focused on inverse dynamic ground reaction force parameters: loading peak force, push-off peak force, peak ratio, impulse, loading rate, and push-off rate parameters. For investigation of gait asymmetry in the selected gait parameters, we chose a symmetry index (SI) commonly used for ground reaction forces sym-metry assessment [16–18].
Measurement instrumentation
The joint kinematic, spatial-temporal, and kinetic data for the intact limb (left leg) and prosthetic limb (right leg) during walking was recorded using the 3D kinematic analyzer CODA Motion System and ODIN software (Charnwood Dynamics Ltd., Leicestershire, England) and a treadmill with force plate—gaitway 3D treadmill, and user software designed by h/p/cosmos (h/p/cosmos Sports & Medical GmbH, Nussdorf, Germany) and by Arsalis (Université catholique de Louvain, Louvain-la-Neuve, Belgium). The gaitway 3D is a fully instrumented electronic single belt treadmill with running space of 150×50 cm including 4 strain gauge sensors measuring all the ground reaction force components during locomotion: the vertical, horizontal, and lateral. As a reliable measuring device with accu-racy of ±2 N, it allows to complex biomechanical pa-rameters during numerous continuous strides of walking to be collected and analyzed in even a limited lab space. Arsalis force amplifier includes 8 force channels with adjustable sensitivity 12–375 mN/bit and sampling rate up to 10 kHz. CODA Motion System is a motion capture system that used 3D active markers and CX1 sensor units with sampling frequency up to 200 Hz. In current study, four CX1 units were used and placed around the treadmill to ensure constant visibility of all active markers during the gait analysis. With a respect to the number of active markers placed on the individual all data were collected in a sampling rate of 200 Hz. Recorded markers data are automatically received by CODAHUB and processed in ODIN software with standard clinical analysis protocol that provides data management and automatically produce standardized gait reports.
Lower body model was defined by markers set placement according to ODIN standard protocol for gait analysis, adapted from previous studies [19–21]. Pelvis, thighs, shanks, knees, ankles and feet were specified by combination of active markers, clusters, and virtual points. The active markers and clusters were placed directly on the lower body segments and ankle-foot prosthesis. The virtual points were defined by pointer stick placement and automatically derived from position of the clusters by the software. Pelvis position was determined by 4 active markers placed on anterior and posterior superior iliac spines. Thigh and shank were determined by clusters: one for the thigh and one for the shank of each side. Each foot was identified by 2 active markers: one on a dorsal aspect of the fifth metatarsal head and one on the upper ridge of the calcaneus posterior surface. Each knee joint was determined by 2 virtual points: one on the medial and one on lateral femoral epicondyle. And, ankle joint of both sides was determined by 2 virtual points: one on medial and one on lateral malleolus. In this case of right ankle-foot prosthesis, pointer was placed to estimated positions of malleoli copying the left intact limb malleoli position.
Measurement procedure
The testing started by clinical assessment of both lower limbs: range of motion of the hip, knee and ankle, leg length and Galeazzi test. Participant had not any other health problem which would influence the gait parameters specifically. Walking motion analysis was performed on 3D treadmill in 5 different walking speeds ranges 2–6 km/h, starting from the slowest speed of 2 km/h gradually rising to the fastest walking speed of 6 km/h. Before the gait test, participant warmed up and got familiar by walking on speed of 1.5 km/h for 3 minutes. Then the speed was increased on 2 km/h and the walking measuring started. Every 2 minutes, the speed was gradually increased by 1 km/h, during contin-uous walking, up to maximal speed of 6 km/h was achieved. After the last test on the speed of 6 km/h, speed was downwardly decreased on 2 km/h for a cool down phase. Minimum of 10 complete gait cycles were recorded for each of limb in all tested speeds after 60 s of speed increasing, in steady state walking. Participant was informed about the increasing the speed approxi-mately 10 s before the increase, but not instructed about the technique or walking style.
Data processing and analysis
The symmetry index (SI) [17, 18] was calculated as the most important determinant for all the selected spatial-temporal and kinetic gait parameters.
where XP is a recorded gait variable for the prosthetic limb and XI is recorded gait variable for the intact limb. An absolute SI value of 5% and higher was considered as a gait asymmetry. Mean of 10 complete gait cycles for the each lower-limb and standard deviation (SD) were calculated automatically in ODIN software for angular range of sagittal plane motion of all the three lower limb joints. Descriptive statistical analysis was processed using Microsoft Excel (MS Office, 2007, USA).
Results
Clinical assessment of the case subject before testing is shown in Table 1.
Results of joint kinematic gait parameters analyses are shown in Table 2.
Data analysis of spatial-temporal and kinetic parame-ters between the slowest (2 km/h) and the fastest (6 km/h) speed is presented in Table 3 and Fig. 1.
Symmetry indexes of joint kinematic, kinetic and spatial-temporal gait parameters are shown in Table 4.
Discussion
In the case study, the prosthetic gait pattern of devel-opmental case of prosthetic gait and gait asymmetry in joints kinematics, spatial-temporal and gait kinetics parameters were investigated in controlled different walking speeds in case of unilateral congenital tibial deficiency. From the results, developmental prosthetic gait pattern was described and gait asymmetry between intact and prosthetic limb was identified for joint kine-matics, spatial-temporal and kinetics parameters during different non-selected walking speeds.
Developmental prosthetic gait pattern of joint kine-matic is presented by increasing mean values of angular range of motion in all joints, while decreasing standard deviations, with increasing gait speed and in both lower limbs. Concretely, joint kinematics shows increasing range of motion in sagittal plane of hip, knee, and ankle with increasing speed for both limbs, except for the prosthetic hip range of motion which increases only non-linearly. However, the initial values of the pros-thetic limb knee and ankle are lower than of the intact limb, except the hip. Recent research of acquired prosthetic gait refer that ankle range of motion is reduced when prosthetic and intact limb compared, and that intact limb has limited ankle range of motion in comparison with able-bodied [5, 10].
Greater hip range of motion in prosthetic limb in our study is probably produced by increasing flexion with faster gait speed, but simultaneously, there is a present decreasing extension, although small. It may be due to weakness of hip extensors muscles and compensatory mechanisms, i.e. restricted ankle plantar-flexion and insufficient push-off force, typically presented in ac-quired prosthetic gait [10].
In the prosthetic limb knee, the clinical assessment showed a neutral knee position of 10° flexion. Thus, the knee may not be enough stable during stance phase as it does not lock into zero extension. This could be reason for compensatory bigger flexion range of the hip, to ensure foot clearance during forward progression, while the push-off force is not adequate in ankle.
These changes may be dependent also on foot pros-thesis type. Their influence on gait characteristics is described by research [7–10]. In one study [22], conven-tional feet with single axis and flex-feet were tested to show ankle’s differences during the early stance phase of prosthetic gait. They founded non-controlled plantar flexion mobility in the conventional foot and decreased plantar flexion mobility in flex-foot type. In other study, authors Barth et al. [8] used motion analysis to test differences between 6 types of foot prostheses and between two groups of traumatic and vascular cases of amputation. They found differences in cadence, veloci-ty, stride length and single limb stance between the 2 groups. Furthermore, step length, late stance ankle dorsal flexion and kinetic parameters were found to differ between six types of prostheses versus intact limb. When we compare mean values of selected gait parame-ters for intact limb, prosthetic limb, and the mean of the both in two opposite extreme speeds of walking—the slowest (2 km/h) and the fastest (6 km/h) speed, we can see that in most of parameters intact limb has higher mean values but also SD in comparison with prosthetic limb, with some exceptions. Primarily, these differences are represented by increasing mean values of each limb separately in the fastest walking speed, except the parameters: push-off peak force, impulse, step duration, and double support duration of the prosthetic limb; and double support duration of the intact limb, which decrease. Concurrently, the mean value of the both limbs increases with the walking speed too, except the impulse and double support duration. Thus, the intact limb is more variable in a compensatory gait pattern in comparison with prosthetic limb. This may be explained by the developmental case of wearing prosthesis, which starts usually in childhood. Thus, the compensatory mechanisms and adaptations to ankle-foot prosthesis progress during longer time and may be qualitatively on better level, in comparison with during life acquired prosthetic gait cases.
Gait asymmetry between intact and prosthetic limb was evaluated using the symmetry index (SI) calcu-lation. Results of SI analysis for joint kinematic parameters show values higher than 5% in parameter total range of motion (ROM) in sagittal plane for all three joints in all tested walking speeds, except hip ROM in speed of 5 km/h. Gait asymmetry is mainly on the intact limb side, except the hip ROM in slower walking speed in which the prosthetic limb presents asymmetry. Thus, the compensatory mechanisms in joint kinematic parameters during prosthetic gait are performed mainly by the intact limb. Furthermore, with increasing speed, the gait asymmetry decreases, except increased ankle ROM asymmetry in the fastest speed of 6 km/h.
Results of SI analysis of spatial-temporal parameters present opposite pattern. Gait asymmetry is mainly on the prosthetic limb side, but only for two (double support duration and step length) from total of 4 parame-ters and only for slower speeds of 2–4 km/h. The com-pensatory mechanisms in spatial-temporal parameters during prosthetic gait are performed mainly by the pros-thetic limb. With increasing speed, the gait asymmetry of spatial-temporal parameters decreases, except the single support duration which almost does not change. Study of acquired prosthetic gait mentions that the stance duration is negatively correlated with walking speed and main reasons included discomfort of weight-bearing on the prosthetic limb and inadequate confi-dence [5]. However, it was not confirmed in our study of developmental case of prosthetic gait. Step duration and single support duration parameters present SI values for intact limb, but lower than 5%, thus not prove the gait asymmetry. It might show that developmental cases of prosthetic gait adapt qualitatively better than during life acquired cases of prosthetic gait and have different gait pattern. Recent research refer that foot prosthesis individuals exhibit longer step length on the prosthetic side compared to the intact side, probably to enhance dynamic stability [5]. Our results confirm this finding, however, just for the slower walking speeds. With in-creasing walking speeds, the gait asymmetry for pros-thetic limb in our study decreases.
SI analysis of kinetic parameters during different speeds presents gait asymmetry only on the intact limb side but in all parameters, except the peak ratio. Only loading rate parameter shows gait asymmetry in all walking speeds while loading peak force, push-off peak force, and push-off rate parameters only in the faster speed of 5–6 km/h and impulse only in the slowest and fastest speed. Additionally, loading peak force, push-off peak force, and push-off rate parameters have clear increasing trend in opposite to loading rate which de-creases, with increasing walking speed. These findings are in accordance with research of acquired prosthetic gait that the intact limb naturally compensates insuf-ficient push-off of passive-elastic ankle-foot prosthetic limb which misses ankle plantar-flexion muscles and tendons, thus insufficiently generate positive power during step-to-step transition [5, 7, 23].
Acquired prosthetic gait research usually measured gait just in self-comfortable speed of walking, in com-parison with our study of gait in different controlled walking speeds. But, acquired prosthetic gait is naturally slower than able-bodied gait. Self-selected amputee gait speed ranges between 1.5 to 2.5 km/h [13] and normal comfortable average speed of gait is approximately 5 km/h [12]. In our study, the gait is all the time mea-sured continuously, just speed is gradually increasing. Thus, this method eliminates the gait variability and asymmetry during acceleration and deceleration phases of gait cycles which arise during level walking in a limit-ed lab space. Furthermore, in this study case, we used 3D treadmill to measure walking in controlled speeds with simultaneous 3D motion capturing. Thus, we may obtain more exact real gait data during different condi-tions, i.e. even non-comfortable walking. Prosthetic gait analysis is mostly performed on the ground walking or walking treadmill [13, 14]. Authors Dingwell et al. [13] show using of walking treadmill for real time visual feedback with defined walking speed for assessment and therapy training of gait asymmetry in TT amputee, but, in self-selected comfortable speed. Other study used treadmill for controlling the speed at the three grades of speed but also, only self-selected speeds [14].
Our results identify developmental prosthetic gait pattern and the gait asymmetry during different walking speeds which demonstrate certain differences in com-parison with during life acquired prosthetic gait pattern. Thus, these findings fill the gap in the prosthetic gait research and may serve as base for further research of developmental cases of prosthetic gait.
Conclusion
Unilateral congenital tibial deficiency is a rare devel-opmental disorder which may lead to prosthetic manage-ment. In the case study, developmental prosthetic gait pattern and the gait asymmetry during different walking speeds were identified. These findings complete the missing point in prosthetic gait research, concretely in developmental case of prosthetic gait and may serve as a base for further research of differences between developmental and acquired cases of prosthetic gait patterns.
Acknowledgement
The work has been supported by UNCE/HUM/032 and SVV 260466.
Phone: +420 605 002 633
Sources
- Fernandez-Palazzi F, Bendahan J, Rivas S. Congenital deficien-cy of the tibia: A report on 22 cases. J Pediatr Orthop Part B. 1998;7(4):298–302. DOI: 10.1097/01202412-199810000-00008
- Tokmakova K, Riddle EC, Kumar SJ. Type IV congenital defi-ciency of the tibia. J Pediatr Orthop. 2003;23(5):649–53.
- Kalamchi A, Dawe RV. Congenital deficiency of the tibia. J Bone Joint Surg Br. 1985;67(4):581–4.
- Litrenta J, Young M, Birch JG, Oetgen ME. Congenital Tibial Deficiency. J Am Acad Orthop Surg. 2019;27:268–79. DOI: 10.5435/JAAOS-D-16-00838
- Richards J. The comprehensive textbook of clincal biome-chanics. 2nd ed. Elsevier Health Sciences; 2018. 1–363 p.
- Torburn L, Perry J, Ayyappa E, Shanfield SL. Below-knee amputee gait with dynamic elastic response prosthetic feet: A pilot study. J Rehabil Res Dev. 1990;27(4):369–84.
- Gitter A, Czerniecki JM, DeGroot DM. Biomechanical analysis of the influence of prosthetic feet on below-knee amputee walking. Am J Phys Med Rehabil. 1991;70(3):142–8. DOI: 10.1097/00002060-199106000-00006
- Barth DG, Schumacher L, Thomas SS. Gait analysis and energy cost of below knee amputeewearing six different prosthetic feet. J Prosthetics Orthot. 1992;4(2):63–75.
- Gage JR, Hicks R. Gait analysis in prosthetics. Clin Prosthetics Orthot. 1985;9:17–23.
- Rietmann JS, Postema K, Geertzen JHB. Gait analysis in prosthetics: opinions, ideas and conclusions. Prosthet Orthot Int. 2002;26:50–7. DOI: 10.1080/03093640208726621
- Isakov E, Keren O, Benjuya N. Trans-tibial amputee gait: Time-distance parameters and EMG activity. Prosthet Orthot Int. 2000;24(3):216–20. DOI: 10.1080/03093640008726550
- Bohannon RW. Comfortable and maximum walking speed of adults aged 20-79 years: reference values and determinants. Age and Ageing. 1997;26:15–19. DOI: 10.1093/ageing/26.1.15
- Dingwell JB, Davis BL, Frazder DM. Use of an instrumented treadmill for real-time gait symmetry evaluation and feedback in normal and trans-tibial amputee subjects. Prosthet Orthot Int. 1996;20(2):101–10. DOI: 10.3109/03093649609164426
- Macfarlane PA, Nielsen DH, Shurr DG, Meier K. Perception of walking difficulty by below-knee amputees using a conventional foot versus the flex-foot. J Prosthetics Orthot. 1991;3(3):114–9.
- Jia X, Wang R, Zhang M, Li X. Influence of prosthetic sagittal alignment on trans-tibial amputee gait and compensating pattern: a case study. Tsinghua Sci Technol. 2008;13(5):581–6. DOI: 10.1016/S1007-0214(08)70095-6
- Sadeghi H, Allard P, Prince F, Labelle H. Symmetry and limb dominance in able-bodied gait: A review. Gait Posture. 2000;12(1):34–45. DOI: 10.1016/S0966-6362(00)00070-9
- Robinson RO, Herzog W, Nigg BM. Use of force platform variables to quantify the effects of chiropractic manipulation on gait symmetry. J Manipulative Physiol Ther [Internet]. 1987 Aug [cited 2019 Mar 26];10(4):172–6. Available from: http://www.ncbi.nlm.nih.gov/pubmed/2958572
- Herzog W, Nigg BM, Read L, Ollson E. Asymmetries in ground reaction force patterns in normal human gait. Med Sci Sport Exerc. 1989;21(1):110–4.
- Bell AL, Pedersen DR, Brand RA. A comparison of the accuracy of several hip center location prediction methods. J Biomech [Internet]. 1990;23(6):617–21.
DOI: 10.1016/0021-9290(90)90054-7 - Leardini A, Cappozzo A, Catani F, Toksvig-Larsen S, Petitto A, Sforza V, et al. Validation of a functional method for the estimation of hip joint centre location. J Biomech. 1999;32:99–103. DOI: 10.1016/S0021-9290(98)00148-1
- Cappozzo A, Catani F, Della Croce U, Leardini A. Position and orientation in space of bones during movement: anatomical frame definition and determination. Clin Biomech. 1995;10(4): 171–8. DOI: 10.1016/0268-0033(95)91394-T
- Rao SS, Boyd LA, Mulroy SJ, Bontrager EL, Gronley JK, Perry J. Segment velocities in normal and transtibial amputees: Pros-thetic design implications. IEEE Trans Rehabil Eng. 1998;6(2): 219–26. DOI: 10.1109/86.681188
- Zelik KE, Adamczyk PG. A unified perspective on ankle push-off in human walking. J Exp Biol. 2016;219(23):3676–83. DOI: 10.1242/jeb.140376
Labels
BiomedicineArticle was published in
The Clinician and Technology Journal
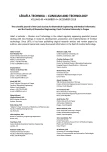
2019 Issue 4
Most read in this issue
- METHODS FOR KINEMATIC ANALYSIS OF HUMAN MOVEMENT IN MILITARY APPLICATIONS: A REVIEW OF CURRENT AND PROSPECTIVE METHODS
- THE EFFECT OF FRAME RATE AND CALIBRATION ON LUNG MONITORING WITH ELECTRICAL IMPEDANCE TOMOGRAPHY
- THE EFFECT OF SPEED ON GAIT ASYMMETRY IN SUBJECT WITH CONGENITAL TIBIAL DEFICIENCY: A CASE STUDY
- THE ADOPTION OF AUTOMATED FiO2 CONTROL INTO POLISH NICUS: 2012–2019