A framework to assess mechanics of stump–socket interaction in transfemoral amputees
Authors:
Alberto Jose Sanchez-Alvarado 1; Vít Nováček 1; Jiří Křen 2
Authors‘ workplace:
New Technologies Research Centre, University of West Bohemia, Pilsen, Czech Republic
1; New Technologies for the Information Society, University of West Bohemia, Pilsen, Czech Republic
2
Published in:
Lékař a technika - Clinician and Technology No. 2, 2019, 49, 46-51
Category:
Original research
Overview
This paper presents the development of a new framework for stump–socket interface force estimation for future prosthesis optimization in transfemoral amputees. It combines experimental data and modelling, including gait analysis, subject-specific simulation of gait and finite element modelling of the residual limb at the prosthesis interaction point. These case-study preliminary results demonstrate how reaction forces between the residual limb and prosthesis can be estimated and used as input data for detailed finite element analysis based on medical images. Gait simulation may provide useful insight in subject-specific musculoskeletal modelling while finite element simulation may provide in-silico feedback for prosthesis optimization with respect to various daily activities.
Keywords:
Gait analysis – Finite element analysis – transfemoral amputees – residual limb–prosthesis interaction
Introduction
Lower-limb amputees present an asymmetrical gait pattern due to the nature of their prosthetic limb, where they usually spend more time during the stance phase on the intact limb and less time on the amputated side, which varies according to the level of amputation and walking speed, as a compensation to protect their residual limb [1]. Moreover, prosthetic components influence the gait parameters, for example, it has been found that a bionic knee improved gait symmetry in transfemoral amputees compared to mechanical knee users [2].
To assess the gait patterns, instrumented gait laboratories integrate different technologies such as electromyography (EMG), segments kinematics (motion capture system) and ground reaction forces (force plates) [3]. These instrumented gait analyses are widely used in several fields such as rehabilitation, wherein patients with an amputation, this tool can be used to assess the rehabilitation program [4], based on the clinical efficacy to quantify functional levels and support clinical decision-making in order to improve the performance due to pathologic conditions [5].
Despite the improvements in gait analysis techniques, this evaluation produces a large amount of data, sometimes of complex interpretation; therefore, gait summary measures have been proposed to quantify the degree of deviation from normal gait pattern [6]. The use of these measures for lower limb amputees has been summarized by Kart et al. [7], where the Gillette Gait Index (GGI), Gait Deviation Index (GDI) and Gait Profile Score (GPS) in conjunction with the Movement Analysis Profile (MAP), have been used. These authors found significant differences between different levels of amputation (transfemoral and transtibial) on the intact side employing a modified version of the GGI and GPS; yet, they also highlighted the need for considering the subject’s functional status, proper marker placement, and prosthetic joint centers, in the analysis of the gait data and use of generic models.
In order to address the implications of generic models, novel simulation software have emerged, where two of the main options are AnyBody™ Modelling System (Anybody Tech, Denmark) and OpenSim (Stanford University, USA) for biomechanical analyses [8], where different scaling, morphing and personalization options can be addressed, based on subject-specific data. Besides, the simulation uses the motion captured data for a parametric optimization of the model and computes the muscle forces, with the possibility to estimate the forces at interest locations of the human–machine interaction.
Multi-body musculoskeletal simulation systems, using inverse dynamics, have recently been used for assessing amputees’ gait patterns [9–11] and other activities such as running [12]. Most of these studies
are based on previous models incorporated into
either AnyBody™ or OpenSim, with customized modifications to these public domain models (Anybody Repository and Hamner model).
Recently, Peng et al. [13] developed a prediction model based on the AnyBody™ Managed Model Repository (AMMR v1.6.2) to compare the simulated knee contact forces and publicly available experimental data from a subject with an instrumented left knee prosthesis [14]; and found a reasonably accuracy
of average root mean square errors (RMSEs) of 0.021×body weight (BW) in the anteroposterior direction, 0.014×BW in the mediolateral direction and 0.089×BW in the vertical direction, employing the Ground Reaction Force Prediction algorithm [15].
On the other hand, related to the prosthetic components, despite of several technological improve-ments, still a large number of amputees have problems with the interaction of the socket and their residual limb, mainly due to sub-optimal design of the socket [16], therefore a “good socket fit” is still unclear for the clinicians, including a limited amount of evidence and the complexity of soft tissue mechanical behavior [17]. Furthermore, high pressures over the skin for long time periods in a non-uniform way can lead to skin ulcers, vascular occlusions, and discomfort, where skin problems can evolve to chronic infections and therefore re-amputation in worst cases [18].
Regarding pressure and stress distribution, Paternò et al. [16] on their review of existing technologies and open challenges for sockets limbs prostheses, highlight that only a few data are available for transfemoral amputees and Finite Elements Analysis (FEA) simula-tions are considered useful tools to optimize the need of extensive measurement on the stump–socket interface. FEA has been successfully employed in simulating the interaction of the residual limb with the prosthetic device [19–21]. More recently, an environment for the simulation-based design of lower limb prosthesis has been developed [22–24]. A similar framework has been developed specifically for selective laser sintering manufacturing technique and transtibial amputees [25].
Despite tremendous progress in computational biomechanics and constantly increasing computational power, most of the scenarios in FEA of residuum–prosthesis interaction is limited to static load evaluating contact pressure and shear stresses at the interface between the tissue and the device. Pre-stress induced in the tissue due to socket donning procedure is also often neglected. It was investigated using an explicit (time-dependent) model for five above-knee amputees [26]. Nevertheless, more research is needed to address clinical controversies and technical difficulties, for a better understanding of comfort and optimal load transfers throughout the gait cycle [18] and other daily life activities.
The main objective of this work is to present the development of a new framework based on and combining gait data from motion capture technology of transfemoral amputees, AnyBody™ modelling to estimate the forces at the socket–residual limb interface, and FEA for detailed analysis of the socket–residual limb interaction in order to provide recommendations with respect to prosthesis optimization.
Methods
Gait Simulation
For the musculoskeletal model, the generic model extracted from AnyBody™ 7.1 AMMR v2.0.0 [27] was used, including the Twenty Lower Extremity Model (TLEM 2.1) [28]. This model was modified excluding all left leg muscles with insertion points or via points lower than an estimated amputation height from the femur segment local coordinate system (5 cm in the direction of the hip joint), i.e. mimicking a transfemoral amputation on the left side.
The simulation was based on a normal gait trial, within the AnyBody™ software. After the removal of the muscles, the segments below amputation level were removed too. Nevertheless, the visualization was kept active for better graphical control. In addition to that, a parameter identification was performed, based on a motion captured data and the inverse dynamics were calculated. In order to estimate the force at the stump–socket interface (created at the amputation height), the forces and moments were transmitted from the simulated stump on to the femur and exported to CSV format. The gait cycle time interval was normalized from 0 to 100%. The forces were normalized to the bodyweight of the generic model (66 kg). Based on the force plate data, the stance phase was defined when the vertical force was greater than 0 N.
Finite Elements Analysis
Finite element model was built including the residual limb, femur, and socket. The geometry (see Fig. 1) was reconstructed from computed tomography (CT) images of a 71 years old male transfemoral amputee. The images were acquired in a supine lying position both without and with the prosthesis. The residual limb and femur were first segmented in ITK-SNAP software [29] and their external envelopes were exported in STL format. MeshLab was used to reduce the number of facets and smooth the surfaces [30]. Both surface meshes were then imported into ANSYS 19.2 SpaceClaim and turned into a 3D geometry. The socket was reconstructed manually from the contours approximated by splines under ANSYS 19.2 Design Modeler and represented by shell elements with 4 mm thickness.
Material properties were adopted from [31]. The socket was considered linearly elastic with Young’s modulus of 1.5 GPa and Poisson’s ratio 0.3. The bone was also considered linearly elastic with Young’s modulus of 15 GPa and Poisson’s ratio 0.3. The residual limb soft tissue was modeled using the Mooney-Rivlin hyperelastic model with C10 = 85.5 kPa, C01 = 21.4 kPa and incompressibility parameter D1 = 1.268 MPa-1 corresponding approximately to Poisson’s ratio 0.459.
Preliminary simulation of the donning procedure was performed using ANSYS 19.2 static solver. The top of the tissue was fixed and frictionless support was defined on the medial plane of the tissue. Displacement towards the tissue was prescribed on the upper part of the socket while displacements in the plane perpendicular to this movement were suppressed to avoid socket rigid body movement before the contact between the socket and residual limb is established. Frictionless contact was defined between the socket and residual limb.
To simulate a steady instant of the gait cycle at the maximum vertical component of the reaction force, a load equivalent to 90 kg (approximate weight of the amputee subject) was applied to the bottom of the socket. The reaction force of the tissue was extracted at the top surface, normalized to the bodyweight and confronted to data from AnyBody™.
Results
Gait Simulation
The simulated gait trial included three steps on force platforms; however, the focus was on the left leg (simulated amputation side corresponding to medical image data available from transfemoral amputee subject) and the duration of the stance phase. Fig. 2 (A) shows the visualization of the simulation after initial left foot contact and contralateral toe-off. The yellow sphere represents the stump–socket interface and the red arrow shows the reaction force vector at this reference point. The blue line represents the ground reaction force vector from the force plate 2. Fig. 2 (B) shows the bodyweight normalized vertical reaction forces at the interface during the stance phase. These forces represent the action of the socket over the stump, i.e. the reaction vector.
Finite Elements Analysis
The FE simulation took less than 13 minutes for a relatively small model with 7261 nodes and 15587 elements (6 core i5-8500 CPU, 16 GB RAM). Strain, stress and contact pressure distributions were as expected and in the usual order of magnitude [21, 26, 31]. Maximum shear stress was less than 75 kPa and contact pressure less than 40 kPa except for few highly localized peaks. FEA results are illustrated in Fig. 1
(D–F).
Further simulation step reproducing the moment of the gait cycle in which the vertical component of the reaction force reached its maximum experienced convergence issues due to contact penetration. For a load equivalent to 90 kg subject, the total body weight normalized peak reaction force was 21.14 N/kg in contrast to 24.86 N/kg provided by AnyBody™.
Discussion
Development of the new framework investigating stump–socket interface mechanics in-silico with future application to prosthesis optimization is presented. It is based on a combination of motion capture gait data, AnyBody™ modelling to estimate the forces at the socket–residual limb interface and FEA for detailed analysis of the socket–residual limb interaction.
Preliminary simulation of a gait cycle in AnyBody™ provided the first estimate of the reaction force at the socket–residual limb interface. Then, this was compared to the corresponding FE simulation incorporating the donning phase to account for pre-stress. Preliminary simulation of the donning procedure demonstrated results close to published data [21, 26, 31] in terms of distributions of stresses, strains, and contact pressure.
The mismatch in reaction force between the two simulations is mostly due to the accepted simpli-fications. The major contribution to this discrepancy is that the FE geometry was reconstructed from MRI images taken in a supine lying position. The geometrical shape of the soft tissue does not correspond to the standing position, leading to an important gap between the socket and the tissue posteriorly. This is where femoral tuberosity plays a crucial role and the contact between the socket and tissue should be well established in this area to ensure stability during gait. Both reaction forces are considerably higher compared to the force measured directly on a volunteer [32]. These authors reported a peak force of less than 800 N for a female amputee (36 years; 1.60 m; 62.65 kg) developed during a standard gait cycle, which represents 30% higher force with respect to the subject’s body weight. Normalized to the bodyweight, this force of 10.77 N/kg would still be lower than computed reactions on our results (FEA: 21.14 N/kg and AnyBody™: 24.86 N/kg). On the other hand, both simulations neglect inertial properties and friction, possibly overestimating the reaction force.
Despite the differences found in vertical peak reaction forces at the socket–stump interface, the waveform profiles (Fig. 2 (B)) show trends similar to published data [32]. Particularly this force presents the expected double peak behavior, demonstrating the applicability of AnyBody™ simulations to estimate forces at the interacttion point of interest. The possibility of force estimation in-silico might lead to better socket design and a “better socket fit”.
Validation of proposed AnyBody™ model simula-tions are anticipated for the next stage, possible options are comparing the onset and offset of muscle activity on the software to measured electromyography on the subjects, similar to [34] or direct comparison of estimated forces to measured forces using an instru-mented prosthesis, similar to [32]. Furthermore, current simulations were based on a non-amputee gait pattern, therefore, data acquisition with amputee subjects is required.
It is expected that, for this project, transfemoral ampu-tees will be recruited in collaboration with clinical partner Protetika Plzeň Ltd. Ethical approval has been recently submitted to the Ethical Committee of New Technologies for the Information Society, University of West Bohemia. The proposed protocol aims to examine various daily activities for selected volunteers using a motion capture system and reaction force measure-ment where appropriate. These data will serve as input
for subject-specific AnyBody™ simulations. By conse-quence, these simulations are expected to provide input data for further detailed FE analysis of the tissue–socket interaction. The novelty of the concept resides in a variety of activities to be examined including dynamic ones, so far ignored by FEA.
It is very unlikely that medical images (CT, MRI, etc.) will be available to construct subject-specific geometry for each subject enrolled in the study. Not only they are costly but are also not suitable because of the acquisition in a supine lying position. It is even more unlikely to identify reliable subject-specific material properties of the tissues. A parametric study is considered a conve-nient strategy to cope with the lack of major FE input data.
Having this in mind, the FE model of the residual limb and socket interaction was simplified in order to run fast and be efficiently used in many runs. The socket geometry has been parametrized via positions of 1080 vertices on its surface.
The tissue geometry will need to be parametrized alike as well as material behavior and coefficients and boundary conditions simulating various daily activities. The ranges of the parameters will need to be defined to cover at least recruited population of amputees. The mechanical outputs (strains, stresses, contact pressures) will need to be carefully related to clinical metrics [33].
The FE model will need to be verified and validated with respect to motion capture and gait analysis data obtained from selected volunteers. Due to lack of subject-specific tissue material data, sensitivity analysis assessing the influence of material behavior and properties will be carried out.
The authors expect that such parametric study, completed by experimental data and AnyBody™ simulations, could reveal major trends and correlations between input and output parameters possibly leading to subject-specific optimization of the prosthetic device and eventually to a proposal of subject-specific rehabili-tation therapy.
Conclusion
A new framework for force estimation at the stump–socket interface for transfemoral amputees that might lead to prosthesis optimization is under development. It combines subject-specific experimental data, medical images and numerical tools including segments kinematics and ground reaction forces measurements in various scenarios, AnyBody™ modelling analyzing these scenarios and predicting the residual limb–socket interface forces, and FEA for detailed analysis of this interaction. Preliminary results are promising and encouraging. Developed tools represent a solid basis for further study on volunteers. It is expected that this framework will reveal major trends and correlations between inputs and outputs possibly leading to recommendations with respect to prosthetic device and rehabilitation program design and, eventually, may serve as clinical decision support.
Acknowledgment
This work was supported by the European Regional Development Fund-Project “Application of Modern Technologies in Medicine and Industry” No. CZ.02.1.01/0.0/0.0/17_048/0007280.
The authors would like to acknowledge Bc. Tomáš Tykal, Bc. Bohumír Chládek and Jan Čarnogurský from Protetika Plzeň Ltd. for their insight in the entire manufacturing process of prosthetic devices and in current customer needs. The authors would also like to acknowledge Ing. Zdeněk Krňoul, Ph.D. and Ing. Pavel Jedlička from New Technologies for the Information Society, the University of West Bohemia for their valuable help and feedback with motion capture.
The authors declare no conflict of interest.
Alberto Jose Sanchez-Alvarado, MSc.
New Technologies Research Centre
University of West Bohemia in Pilsen, CZ
E-mail: sancheza@ntc.zcu.cz
Sources
- Nolan L, Wit A, Dudzinski K, Lees A, Lake M, Wychowanski M. Adjustments in gait symmetry with walking speed in trans-femoral and trans-tibial amputees. Gait & Posture. 2003 Apr; 17(2):142–51.
- Uchytil J, Jandacka D, Zahradnik D, Farana R, Janura M. Temporal–spatial parameters of gait in transfemoral amputees: Comparison of bionic and mechanically passive knee joints. Prosthet Orthot Int. 2014 Jun; 38(3):199–203.
- Vaughan C, Davis B, O’Connor J. Dynamics of Human Gait. Cape Town: Kiboho Publishers; 1999.
- Kingsbury T, Marks M, Thesing N, Myers G, Isken M, Wyatt M. Use of an Amputee Gait Score to Assess Rehabilitation Progress. 37th Annual Meeting of the American Society of Biomechanics. 2013 Sep 4–7; Omaha, NE, USA.
- Cimolin V, Condoluci C, Costici P, Galli M. A proposal for a kinetic summary measure: the Gait Kinetic Index. Comput Methods in Biomech Biomed Engin. 2018 Nov 16; 1–6.
- Cimolin V, Galli M. Summary measures for clinical gait analysis: A literature review. Gait Posture. 2014 Apr; 39(4): 1005–10.
- Kark L, Vickers D, McIntosh A, Simmons A. Use of gait summary measures with lower limb amputees. Gait Posture. 2012 Feb; 35(2):238–243.
- Trinler U, Schwameder H, Baker R, Alexander N. Muscle force estimation in clinical gait analysis using AnyBody and OpenSim. J Biomech. 2019 Mar 27; 86:55–63.
- Voinescu M, Soares D, Jorge RN, Davidescu A, Machado L. Estimation of the forces generated by the thigh muscles for transtibial amputee gait. J Biomech. 2012 Apr 5; 45(6):972–7.
- Schwarze M, Hurschler C, Seehaus F, Oehler S, Welke B. Loads on the prosthesis–socket interface of above-knee amputees during normal gait: Validation of a multi-body simulation. J Biomech. 2013 Apr 5; 46(6):1201–6.
- Salami F, Heitzmann D, Leboucher J, Alimusaj M, Wolf S. P 122 - Modelling of residual limb and socket interaction in individuals with trans-femoral prosthesis during walking. Gait & Posture. 2018 Sep; 65:440–1.
- Kersting UG, Gurney JK, Lewis S, Rasmussen J. An individualized musculoskeletal model for the analysis
of amputee running. 13th International Symposium of Biomechanics in Sports. 2010 Jul 19–23; Marquette, MI, USA. 142–4. - Peng Y, Zhang Z, Gao Y, Chen Z, Xin H, Zhang Q, Fan X, Jin Z. Concurrent prediction of ground reaction forces and moments and tibiofemoral contact forces during walking using musculoskeletal modelling. Med Eng. Phys. 2018 Feb; 52: 31–40.
- Fregly BJ, Besier TF, Lloyd DG, Delp SL, Banks SA, Pandy MG, et al. Grand challenge competition to predict in vivo knee loads. J Orthop Res. 2012 Apr; 30(4):503–13.
- Fluit R, Andersen MS, Kolk S, Verdonschot N, Koopman HF. Prediction of ground reaction forces and moments during various activities of daily living. J Biomech. 2014 Jul 18; 47(10):2321-9.
- Paternò L, Ibrahimi M, Gruppioni E, Menciassi A and Ricotti L. Sockets for limb prostheses: a review of existing technologies and open challenges. IEEE Trans Biomed Eng. 2018 Sep; 65(9):
1996–2010. - Dumbleton T, Buis AW, McFadyen A, McHugh BF, McKay G, Murray KD, et al. Dynamic interface pressure distributions of two transtibial prosthetic socket concepts. J Rehabil Res Dev. 2009; 46(3):405–15.
- Mak AF, Zhang M, Boone DA. State-of-the-art research in lower-limb prosthetic biomechanics-socket interface: a review. J Rehabil Res Dev. 2001 Mar-Apr; 38(2):161–74.
- Zhang M, Mak AF, Roberts VC. Finite element modelling of a residual lower-limb in a prosthetic socket: a survey of the development in the first decade. Med Eng Phys. 1998 Jul; 20(5):360–73.
- Silver-Thorn MB, Steege JW, Childress DS. A review of prosthetic interface stress investigations. J Rehabil Res Dev. 1996 Jul; 33(3):253–66.
- Dickinson AS, Steer JW, Worsley PR. Finite element analysis
of the amputated lower limb: a systematic review and recommendations. Med Eng Phys. 2017 May; 43: 1–18. - Colombo G, Facoetti G, Morotti R, Rizzi C. Physically based modelling and simulation to innovate socket design. Computer-Aided Design and Applications. 2011 Jan 1; 8(4):617–31.
- Colombo G, Facoetti G, Rizzi C. A digital patient for computer-aided prosthesis design. Interface focus. 2013 Apr 6; 3(2):
20120082. - Colombo G, Rizzi C, Regazzoni D, Vitali A. 3D interactive environment for the design of medical devices. IJIDeM. 2018 May; 12(2):699–715.
- Faustini MC, Neptune RR, Crawford RH, Rogers WE, Bosker G. An experimental and theoretical framework for manufacturing prosthetic sockets for transtibial amputees. IEEE Transactions on Neural Systems and Rehabilitation Engineering. 2006 Sep; 14(3):304–10.
- Lacroix D, Patiño JF. Finite element analysis of donning procedure of a prosthetic transfemoral socket. Ann Biomed Eng. 2011 Dec; 39(12):2972–83.
- Damsgaard M, Rasmussen J, Christensen ST, Surma E, de Zee M. Analysis of musculoskeletal systems in the AnyBody Modelling System. Simulation Modelling Practice and Theory. 2006; 14(8):1100–11.
- Klein Horsman MD, Koopman HF, van der Helm FC, Prosé LP, Veeger HE. Morphological muscle and joint parameters for musculoskeletal modelling of the lower extremity. Clin Biomech (Bristol, Avon). 2007 Feb; 22(2):239–47.
- Yushkevich PA, Piven J, Hazlett HC, Smith RG, Ho S, Gee JC, et al. User-guided 3D active contour segmentation of anatomical structures: Significantly improved efficiency and reliability. Neuroimage. 2006 Jul 1; 31(3):1116–28.
- Cignoni P, Callieri M, Corsini M, Dellepiane M, Ganovelli F, Ranzuglia G. MeshLab: an open-source mesh processing tool. Sixth Eurographics Italian Chapter Conference. 2008 Jul 2–4; Salerno, Italy. 129–36.
- Zhang L, Zhu M, Shen L, Zheng F. Finite element analysis of the contact interface between trans-femoral stump and prosthetic socket. 35th annual international conference of the IEEE EMBS. 2013 Jul 3-7; Osaka, Japan. 1270–3.
- Frossard LA, Beck J, Dillon M, Evans JH. Development and preliminary testing of a device for the direct measurement of forces and moments in the prosthetic limb of transfemoral amputees during activities of daily living. J Prosthet Orthot. 2003 Oct; 15(4):135–42.
- Portnoy S, Siev-Ner I, Yizhar Z, Kristal A, Shabshin N, Gefen A. Surgical and morphological factors that affect internal mechanical loads in soft tissues of the transtibial residuum. Ann Biomed Eng. 2009 Dec; 37(12):2583–605.
- Wibawa A, Verdonschot N, Burgerhof J, Purnama I, Andersen M, Halbertsma J, et al. A validation study on muscle activity prediction of a lower limb musculoskeletal model using EMG during normal walking. 2013 3rd International Conference on Instrumentation, Communications, Information Technology and Biomedical Engineering (ICICI-BME). 2013.
Labels
BiomedicineArticle was published in
The Clinician and Technology Journal
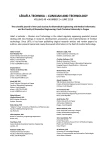
2019 Issue 2
Most read in this issue
- Effect of the zinc phthalocyanine mediated photodynamic therapy on cytoskeletal apparatus of hela cells
- Methods evaluating upper arm and forearm movement during a quiet stance
- Wrist rehabilitation with manipulator to perform passive and active exercises
- A framework to assess mechanics of stump–socket interaction in transfemoral amputees